As the 5G era dawns, the need for robust transport network architectures has never been more critical. The advent of 5G brings with it a promise of unprecedented data speeds and connectivity, necessitating a backbone capable of supporting a vast array of services and applications. In this realm, the Optical Transport Network (OTN) emerges as a key player, engineered to meet the demanding specifications of 5G’s advanced network infrastructure.
Understanding OTN’s Role
The 5G transport network is a multifaceted structure, composed of fronthaul, midhaul, and backhaul components, each serving a unique function within the overarching network ecosystem. Adaptability is the name of the game, with various operators customizing their network deployment to align with individual use cases as outlined by the 3rd Generation Partnership Project (3GPP).
C-RAN: Centralized Radio Access Network
In the C-RAN scenario, the Active Antenna Unit (AAU) is distinct from the Distribution Unit (DU), with the DU and Central Unit (CU) potentially sharing a location. This configuration leads to the presence of fronthaul and backhaul networks, and possibly midhaul networks. The fronthaul segment, in particular, is characterized by higher bandwidth demands, catering to the advanced capabilities of technologies like enhanced Common Public Radio Interface (eCPRI).
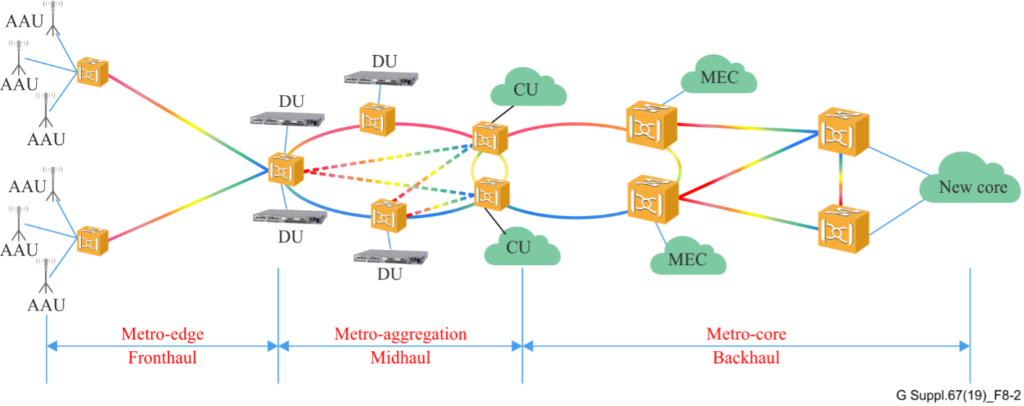
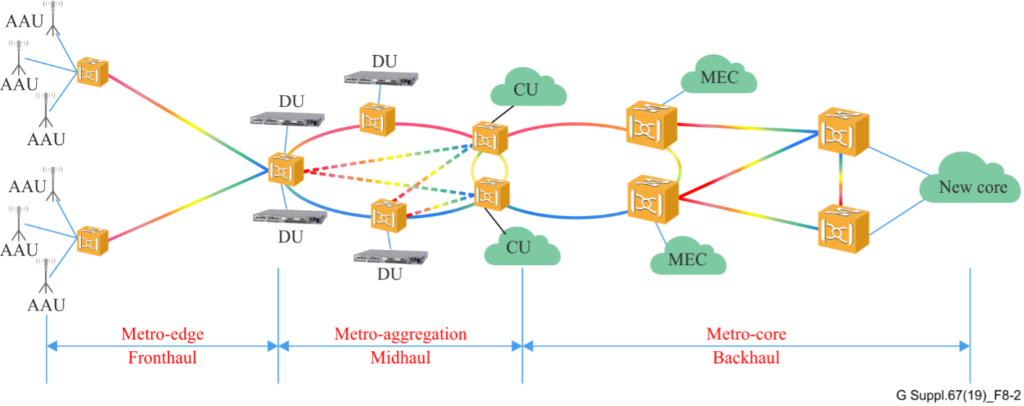
C-RAN Deployment Specifics:
- Large C-RAN: DUs are centrally deployed at the central office (CO), which typically is the intersection point of metro-edge fibre rings. The number of DUs within in each CO is between 20 and 60 (assume each DU is connected to 3 AAUs).
- Small C-RAN: DUs are centrally deployed at the metro-edge site, which typically is located at the metro-edge fibre ring handover point. The number of DUs within each metro-edge site is around 5~10
D-RAN: Distributed Radio Access Network
The D-RAN setup co-locates the AAU with the DU, eliminating the need for a dedicated fronthaul network. This streamlined approach focuses on backhaul (and potentially midhaul) networks, bypassing the fronthaul segment altogether.
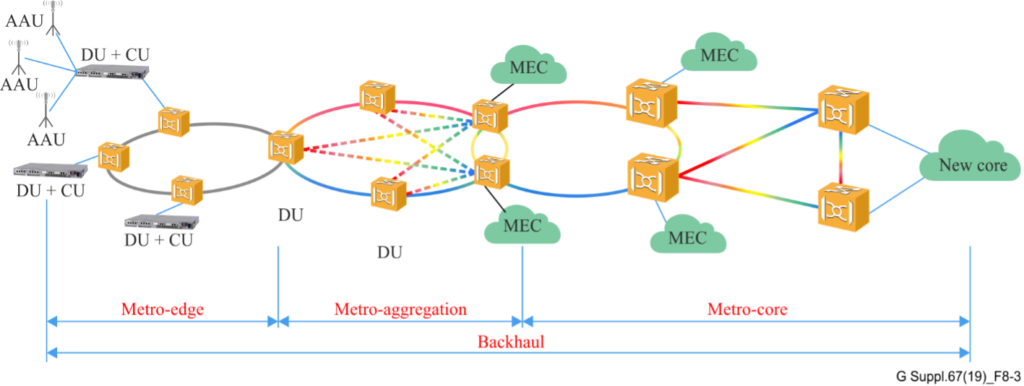
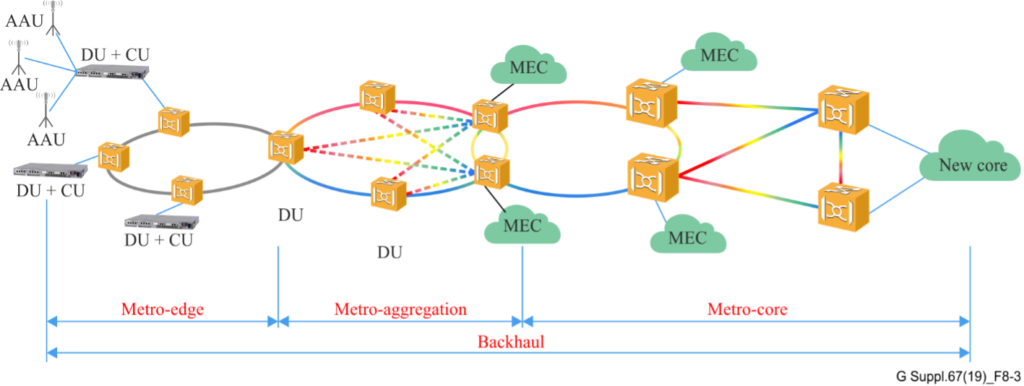
NGC: Next Generation Core Interconnection
The NGC interconnection serves as the network’s spine, supporting data transmission capacities ranging from 0.8 to 2 Tbit/s, with latency requirements as low as 1 ms, and reaching distances between 100 to 200 km.
Transport Network Requirement Summary for NGC:
Parameter | Requirement | Comments |
---|---|---|
Capacity | 0.8-2 Tbit/s | Each NGC node has 500 base stations. The average bandwidth of each base station is about 3Gbit/s, the convergence ratio is 1/4, and the typical bandwidth of NGC nodes is about 400Gbit/s. 2~5 directions are considered, so the NGC node capacity is 0.8~2Tbit/s. |
Latency | 1 ms | Round trip time (RTT) latency between NGCs required for DC hot backup intra-city. |
Reach | 100-200 km | Typical distance between NGCs. |
Note: These requirements will vary among network operators.
The Future of 5G Transport Networks
The blueprint for 5G networks is complex, yet it must ensure seamless service delivery. The diversity of OTN architectures, from C-RAN to D-RAN and the strategic NGC interconnections, underscores the flexibility and scalability essential for the future of mobile connectivity. As 5G unfolds, the ability of OTN architectures to adapt and scale will be pivotal in meeting the ever-evolving landscape of digital communication.