DWDM Practical guide for cracking optical interviews |
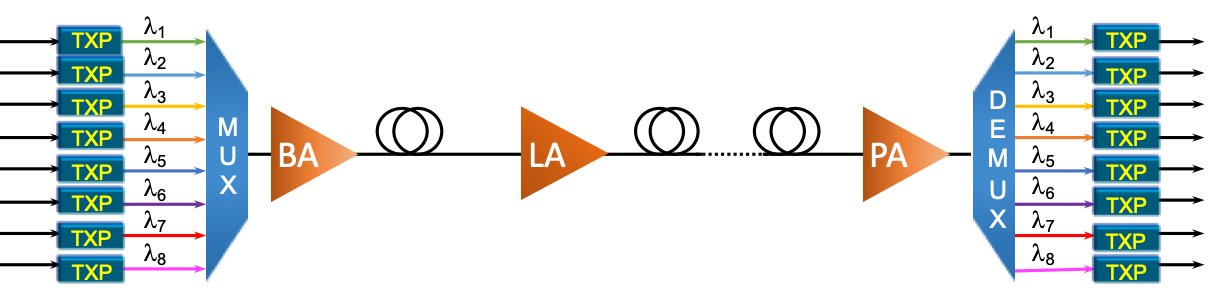
INTRODUCTION
MapYourTech’s Interview Buddy Series is an initiative to help Optical Fiber Communication Professionals increase their technical and behavioral interview skill sets which will help them excel in their professional career.
In this series, utmost care has been taken to include practical DWDM based questions that are asked in related industries during current time.
Intent is to enable optical professionals’ interest and equipping them with right tools to excel in their career.
DWDM (Dense Wavelength Division Multiplexing) is an interesting branch of Optical Fiber Communication which acts as a backbone to the telecom networks delivering high capacity and highspeed data from one end to another.
TABLE OF CONTENT
Question 1: What is DWDM and how it works?
Question 2: What are the basic component of DWDM link?
Question 3: What is a transponder?
Question 4: What are the major types of transponder used in network?
Question 5: What are the major advantages of using coherent transponders?
Question 6: What are performance parameters on Transponders?
Question 7: What is a multiplexer (MUX) and a demultiplexer(DEMUX)?
Question 8: What is an Amplifier ? What are the types of amplifier we use in a DWDM Network ?
Question 10: What is ASE and how does it affect EDFA performance?
Question 11: What is the maximum number of EDFA’s to cascade in a DWM link and why?
Question 12: What are main advantages and drawbacks of EDFAs?
Question 13: What are the types of Amplifiers based on their placement in a DWDM Link?
Question 14: What is power control mode and Gain control mode?
Question 15: What is the effect of Amplifier gain on OSNR while reducing or increasing flat gain?
Question 16: What is gain tilt and Gain ripple?
Question 17: What is a 30 dB gain means?
Question 18: What is Raman Amplifier and how does it work?
Question 19: What are the advantages of using Raman Amplifier?
Question 20: What are the Noise sources in Raman Amplifier?
Question 22: Why generally EDFA and Raman are used in conjunction to each other?
Question 23: What are linear effects?
Question 24: What are nonlinear effects?
Question 25: What are the types of Nonlinear effects that happens in a DWDM link?
Question 26: What is impact of linear and nonlinear effects in DWDM network?
Question 27: How to reduce FWM impact?
Question 29: What are different types of fibers and what is their significance?
Question 30: What are Fiber spectrum bands?
Question 31: What is Red and Blue and Red Band is preferred over Blue in DWDM?
Question 32: What is dark fiber, dim fiber and lit fiber?
Question 33: What are the sources of latency in optical fiber?
Question 34: What is micro bending and macro bending?
Question 35: What is fiber characterization?
Question 37: What is PMD coefficient and its unit?
Question 40: What is cause of CD?
Question 41: What is unit of CD and CD Coefficient?
Question 42: Which are main factors for CD?
Question 43: How to mitigate CD in a link?
Question 44: What are general performance parameters available on an Optical Amplifiers?
Question 47: What are methods to reduce CD in link?
Question 48: What is DCM or DSCM or DCU?
Question 49: What are considerations while deploying DCM?
Question 50: What are the types of DCM in DWDM link?
Question 53: What is Optical Return Loss (ORL) in Optical Fiber system?
Question 54: What are the major sources of ORL?
Question 55: what are the implications of ORL?
Question 56: How does reflected power affect laser stability?
Question 58: What are the methods to help improve ORL?
Question 59: Why it is Good to have ORL >30dB for general fiber links?
Question 61: What is Q-factor and what is its importance?
Question 62: What are the advantages of Coherent Optical Transmission System?
Question 63: Why Receiver Sensitivity is so important for optical module?
Question 64: What is attenuation in Optical fiber?
Question 65: What are the general colors of patch cord seen in Optical environment?
Question 66: Defining Colorless, Directionless, Contention-less flexible grid Network?
Question 67: Why Do We Need Gridless?
Question 68: What Is Coherent Communication?
Question 70: What does Tap ration means for splitter and Coupler?
Question 71: What an OTDR can do for you?
Question 72: What is the difference between HD-FEC & SD-FEC in coherent transponders?
Question 73: Why is it preferable to put attenuator/pad at the Receive end of Optical Module?
Question 74: How and where do we get pre and post FEC BER?
Question 75: what are the Optical Fiber Link Design requirements?
Question 77: What is the relationship between BER and Q factor?
Question 78: What are the noise sources known in Optical fiber network?
Question 79: What is resolution bandwidth?
Question 80: What is noise equivalent bandwidth?
Question 82: Why is the BER not easy to simulate/calculate?
Question 83: What is ROADM? What problems ROADM can solve?
Question 84: What is TVSP, and what is its effect?
Question 85: What are the main reasons behind the fluctuation of the Q factor?
Question 86: What is Spectral Efficiency, and what is its role in coherent technologies?
Question 87: What is the basic behind increasing SE?
Question 88: What is the main purpose of using Coherent Detection in a system?
Question 89: How does changing modulation improves the reach of a system?
Question 90: What are the key characteristics of optical amplifiers?
Question 91: What are the main issues associated with EDFA in a DWDM link?
Question 92: What are the parameters associated with fibers in a link?
Question 93: What are the parameters associated with optical light sources in a link?
Question 94: What are the parameters associated with optical light receivers in a link?
Question 95: How does temperature affects EDFA performance?
Question 96: What are some ways to increase the capacity of an optical system?
Question 97: What is the significance of the eye diagram?
Question 99: Why does FEC introduce latency?
Question 101: What is dBm and what are the major conversions used in Optical Network?
Question 102: Which components and technology is used in ROADM?
This page is left intentionally blank
Question 1: What is DWDM and how it works?
Dense Wavelength division multiplexing (DWDM) is a technology used to combine or retrieve two or more optical signals of different optical center wavelengths or frequencies in a fiber. This allows fiber capacity to be expanded in the frequency domain from one channel to greater than 100 channels. This is accomplished by first converting standard, non-DWDM optical signals to signals with unique WDM wavelengths or frequencies that will correspond to the available channel center wavelengths in the WDM multiplexer and demultiplexer. Typically, this is done by replacing non-WDM transceivers with the proper WDM channel transceivers. WDM channels are defined and labeled by their center wavelength or frequency and channel spacing. The WDM channel assignment process is an industry standard defined in International Telecommunications Union (ITU-T). Then different WDM signal wavelengths are combined over one fiber by the WDM multiplexer. In the fiber, the individual signals propagate with minimal interaction assuming low signal power. For high powers, multiple interactions can occur. Once the signals reach the fiber link end, the WDM demultiplexer separates the signals by their wavelengths, back to individual fibers that are connected to their respective equipment receivers. Optical receivers have a broad reception spectrum, which includes all of C band. Many receivers can also receive signals with wavelengths down to O band.
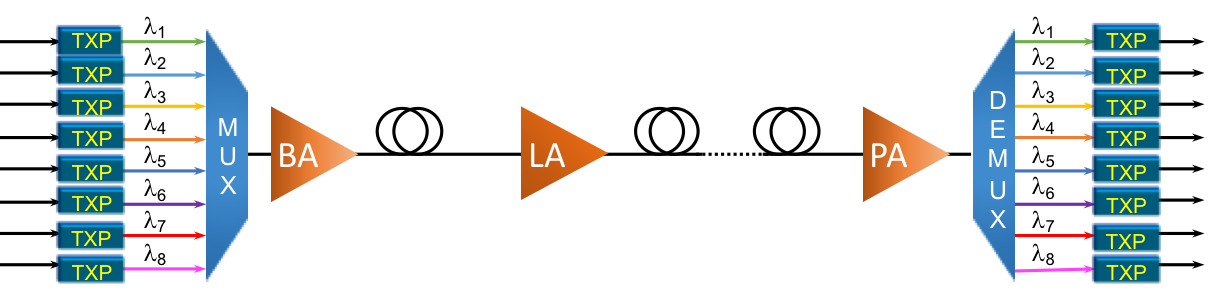
Above schematics show basic DWDM block diagram.
Question 2: What are the basic component of DWDM link?
DWDM components includes: –
Transponders
To convert grey or black and white signals to colored (different frequency) signals with O-E-O mechanism.
Multiplexer
To aggregate different channels in form of composite channel.
Amplifier
To boost signal strength so that it can travel large distance.
Demultiplexer
To dis-aggregate various channel coming from network to respective frequencies.
Question 3: What is a transponder?
Transponder is the integrated part of WDM systems use to transmit signal over a DWDM link. This module takes black and white or grey signals as input on 1310nm, 1550nm or 850nm and converts those signals into colored channels or certain frequencies in C or L band. This is achieved by using optical-electrical-optical conversion mechanism. Transponders along with Optical source also includes complex components that helps signal in serialization and deserialization of frames, control and monitoring capabilities etc.
There are two types of transponders:
• optical – to – electrical – to – optical (O – E – O)
• optical – to – optical (O – O).
The O – E – O transponder may also act as a 3R repeater; that is, it performs signal reshaping, retiming, and reconstitution or gain; O – E – Os are more complex and more expensive, Because the signal is converted to electronic, an O – E – O node allows for add – drop functionality, in addition to simple optical relay or transponder.
The O – O transponder, or optical relay, is technologically more attractive because it performs direct optical – to – optical amplification using optical amplifiers (doped fiber – based (EDFA) or semiconductor optical amplifiers (SOA)) thus acting as an all – optical relay.
Question 4: What are the major types of transponder used in network?
There are two types of transponders
• non coherent transponders
• coherent transponders
non coherent transponders:
These transponders involve IM/DD (Intensity Modulation/Direct Detection) technique also known as OOK method for transmission of signal. In IM/DD the intensity, or power, of the light beam from a laser or a light-emitting diode (LED) is modulated by the information bits and no phase information is needed. Due to this nature, no local oscillator is required for IM/DD communication, which greatly eases the cost of the hardware.
coherent transponders:
The basic idea behind coherent detection consists of combining the optical signal coherently with a continuous-wave (CW) optical field before it falls on the photodetector. The CW field is generated locally at the receiver using a narrow line width laser, called the local oscillator (LO). With the mixing of the received optical signal with the LO output can improve the receiver performance.
Question 5: What are the major advantages of using coherent transponders?
The major advantage of using the coherent detection techniques is that both the amplitude and the phase of the received optical signal can be detected, extracted and measured accordingly. This method helps in sending information by modulating either the amplitude, or the phase, or the frequency of an optical carrier. In the case of digital communication systems, the three possibilities give rise to three modulation formats known as amplitude-shift keying (ASK), phase-shift keying (PSK), and frequency-shift keying (FSK)
Use of coherent detection may allow a more efficient use of fiber bandwidth by increasing the spectral efficiency of WDM system. Sometimes it has been seen that the receiver sensitivity can be improved by up to 20 dB compared with that of IM/DD systems BER, and hence the receiver sensitivity.
Question 6: What are performance parameters on Transponders?
Usually Transponders have following optical parameters to monitor:
Optical power receives. |
Normalized optical power receive. |
Optical power receive (minimum). |
Optical power receive (maximum). |
Optical power receive (average). |
Optical power transmit. |
Optical power transmit (minimum). |
Optical power transmit (maximum). |
Optical power transmit (average). |
Optical power receive OTS. |
Normalized optical power receive OTS. |
Optical power receive OTS (minimum). |
Optical power receive OTS (maximum). |
Optical power receive OTS (average). |
Differential group delay (average). |
Differential group delay (maximum). |
Code violations, OTU, near end receive. |
Errored seconds, OTU, near end receive. |
Severely errored seconds, OTU, near end receive. |
Severely errored frame seconds, OTU, near end receive. |
FEC corrections, OTU, near end receive. |
High correction count seconds, OTU, near end receive. |
Pre-FEC BER, OTU, near end receive. |
Pre-FEC BER (maximum), OTU, near end receive. |
Post-FEC BER estimates, OTU, near end receive. |
Q min, OTU, near end receive. This represents the Q low water mark. |
Q max, OTU, near end receive. This represents the Q high water mark. |
Q average, OTU, near end receive. This represents the average Q during the measured interval. |
Q standard deviation, OTU, near end receive. This represents the standard deviation of the Q during the measured interval. |
Uncorrected FEC block, OTU, near end receive. |
Code violations, ODU, near end receive. |
Errored seconds, ODU, near end receive. |
Severely errored seconds, ODU, near end receive. |
Unavailable seconds, ODU, near end receive. |
Failure count, ODU, near end receive. |
Question 7: What is a multiplexer (MUX) and a demultiplexer(DEMUX)?
As DWDM systems send signals from several sources over a single fiber, they must be able to combine the incoming signals. This is done with a multiplexer, which takes optical wavelengths from multiple fibers and converges them into one beam. At the receiving end, the system must be able to separate out the components of the light so that they can be discreetly detected. Demultiplexers perform this function by separating the received beam into its wavelength components and coupling them to individual fibers. Demultiplexing must be done before the light is detected, because photo-detectors are inherently broadband devices that cannot selectively detect a single wavelength.
Multiplexers and demultiplexers can be either passive or active in design. Passive designs are based on prisms, diffraction gratings or filters, while active designs combine passive devices with tunable filters. The primary challenges in these devices is to minimize cross-talk and maximize channel separation. Cross-talk is a measure of how well the channels are separated, while channel separation refers to the ability to distinguish each wavelength
Question 8: What is an Amplifier ? What are the types of amplifier we use in a DWDM Network ?
Amplifiers are the modules used generally in long haul networks to manage loss in a DWDM network. Here the signal is directly amplified without conversion of optical signal into electrical signal .
Optical Amplifiers amplify input light through stimulated emission, the same mechanism that is used by lasers but only difference is that amplifiers doesn’t need feedback circuitry. It’s main ingredient is the optical gain realized when the amplifier is pumped (electrically or optically ) to achieve population inversion. The optical gain, in general, depends not only on the frequency (or wavelength) of the incident signal, but also on the local signal intensity at any point inside the amplifier.
There are mainly two types of amplifiers used in DWDM network and they are EDFA(Erbium doped fiber Amplifier ) and Raman Amplifier.
EDFA:
Erbium doped fiber amplifiers makes use of rare-earth elements (Er3+ ) as a gain medium by doping the fiber core during the manufacturing process .Erbium-doped fiber amplifiers (EDFAs) is widely used because they operate in the wave- length region near 1.55 μm .In EDFA,pumping at a suitable wavelength provides gain through population inversion. The gain spectrum depends on the pump- ing scheme as well as on the presence of other dopants, such as germania and alumina, within the fiber core. Efficient EDFA pumping is possible using semiconductor lasers operating near 0.98- and 1.48-μm wavelengths. Most EDFAs use 980-nm pump lasers as such lasers are commercially available and can provide more than 100 mW of pump power. Pumping at 1480 nm requires longer fibers and higher powers because it uses the tail of the absorption band ,
RAMAN:
Raman fiber uses SRS (stimulated Raman scattering ) phenomenon which was experimentally observed by Sir Chandrasekhara Venkata Raman in 1928.
SRS is used in silica fibers when an intense pump beam propagates through it .With this effect the incident pump photon gives up its energy to create another photon of reduced energy at a lower frequency (inelastic scattering); the remaining energy is absorbed by the medium in the form of molecular vibrations (optical phonons). Thus, Raman amplifiers must be pumped optically to provide gain. The pump and signal beams at different frequencies are injected into the fiber through a fiber coupler. The energy is transferred from the pump beam to the signal beam through SRS as the two beams co-propagate inside the fiber. Commonly counter propagation mode is used. The gain from a Raman amplifier increases almost linearly with the wave- length offset between signal and pump, peaking at about an 100-nm difference, then it drops off rapidly.
Question 9: What is the difference between 980nm and 1480nm pump based Erbium Doped Fiber Amplifiers (EDFA)?
The 980nm pump needs three energy level for radiation while 1480nm pumps can excite the ions directly to the metastable level .
Though pumping with 1480 nm is used and has an optical power conversion efficiency which is higher than that for 980 nm pumping, the latter is preferred because of the following advantages it has over 1480 nm pumping.
▪ It provides a wider separation between the laser wavelength and pump wavelength.
▪ 980 nm pumping gives less noise than 1480nm.
▪ Unlike 1480 nm pumping, 980 nm pumping cannot stimulate back transition to the ground state.
▪ 980 nm pumping also gives a higher signal gain, the maximum gain coefficient being 11 dB/mW against 6.3 dB/mW for the 1.48
▪ The reason for better performance of 980 nm pumping over the 1.48 m pumping is related to the fact that the former has a narrower absorption spectrum.
▪ The inversion factor almost becomes 1 in case of 980 nm pumping whereas for 1480 nm pumping the best one gets is about 1.6.
▪ Quantum mechanics puts a lower limit of 3 dB to the optical noise figure at high optical gain. 980 nm pimping provides a value of 3.1 dB, close to the quantum limit whereas 1.48 pumping gives a value of 4.2 dB.
▪ 1480nm pump needs more electrical power compare to 980nm.
▪ Typically, 980 nm pumping results in a noise figure 1 dB lower than that for 1480 nm pumping.
▪ The shorter wavelength results in less noise.
Question 10: What is ASE and how does it affect EDFA performance?
During population inversion phenomenon and as spontaneous emission occurs in all modes supported by the fiber (guided and unguided). Clearly, some of these photons would appear from time to time in the same fiber mode occupied by the signal field. Such spontaneously emitted photon perturbs both the amplitude and the phase of the optical field in a random fashion. These random perturbations of the signal are the source of amplifier noise in EDFAs and results in ASE.
For long haul links, generally EDFA’s are cascaded to overcome fiber losses in the link. Due to these cascading structures, amplifier induced noise buildup and impacts the performance of Amplifier. The ASE accumulates over many amplifiers and degrades the optical SNR. Also, as the level of ASE grows, it begins to saturate optical amplifiers and reduce the gain of amplifiers located further down the fiber link. The net result is that the signal level drops further while the ASE level increases. So, it’s obvious that if the number of amplifiers is large, the SNR will degrade so much at the receiver that the BER will become unacceptable.
Question 11: What is the maximum number of EDFA’s to cascade in a DWM link and why?
Maximum number of erbium-doped fiber amplifiers (EDFAs) in a fiber chain is about four to six.
The rule is based on the following rationales:
1. About 80 km exists between each in-line EDFA, because this is the approximate distance at which the signal needs to be amplified.
2. One booster is used after the transmitter.
3. One preamplifier is used before the receiver.
4. Approximately 400 km is used before an amplified spontaneous emission (ASE) has approached the signal (resulting in a loss of optical signal-to-noise ratio [OSNR]) and regeneration needs to be used.
An EDFA amplifies all the wavelengths and modulated as well as unmodulated light. Thus, every time it is used, the noise floor from stimulated emissions rises. Since the amplification actually adds power to each band (rather than multiplying it), the signal-to-noise ratio is decreased at each amplification. EDFAs also work only on the C and L bands and are typically pumped with a 980- or 1480-nm laser to excite the erbium electrons. About 100 m of fiber is needed for a 30-dB gain, but the gain curve doesn’t have a flat distribution, so a filter is usually included to ensure equal gains across the C and L bands.
Question 12: What are main advantages and drawbacks of EDFAs?
Advantages:
▪ Commercially available in C band (1,530 to 1,565 nm) and L band (1,560 to 1,605) and up to 84-nm range at the laboratory stage.
▪ Excellent coupling: The amplifier medium is an SM fiber;
▪ Insensitivity to light polarization state;
▪ Low sensitivity to temperature;
▪ High gain: > 30 dB with gain flatness < ±0.8 dB and < ±0.5 dB in C and L band, respectively, in the scientific literature and in the manufacturer documentation
▪ Low noise figure: 4.5 to 6 dB
▪ No distortion at high bit rates;
▪ Simultaneous amplification of wavelength division multiplexed signals;
▪ Immunity to crosstalk among wavelength multiplexed channels (to a large extent)
Drawbacks:
▪ Pump laser necessary;
▪ Difficult to integrate with other components;
▪ Need to use a gain equalizer for multistage amplification;
▪ Dropping channels can give rise to errors in surviving channels: dynamic control of amplifiers is necessary.
Question 13: What are the types of Amplifiers based on their placement in a DWDM Link?
With the placements, there are three types of amplifiers:
• Booster Amplifier
• Pre-Amplifier
• In-Line Amplifier
Booster Amplifier
Main purpose of booster amplifier is to boost the power transmitted.
A booster amplifier is used to amplify the signal channels exiting the transmitter to the level required for launching into the fiber link. In most applications this level is in the range of 0-5 dBm per channel, however, it can be higher for more demanding applications. A booster is not always required in single channel links, but is essential in a WDM link where the multiplexer attenuates the signal channels. A booster amplifier typically has low gain (in the range of 5-15 dB) and high output power, typically about 20dBm for a 40 channel WDM system. The NF of a booster amplifier is not usually a critical parameter. At the other end of a link a pre-amplifier may be required to amplify the optical signal to the level where it can be detected over and above the thermal noise of the receiver.
Pre-Amplifier
These amplifiers are commonly used to improve the receiver sensitivity. Transmission distance can also be increased by putting an amplifier just before the receiver to boost the received power.
A pre-amplifier should provide high gain, often in the range of 30 dB, and have a low NF in the range of 4-5.5 dB, in order to assure error-free detection of the signal channels. The output power of the pre-amplifier need not be very high.
For links up to about 150 km, a booster and/or pre-amplifier are usually sufficient to ensure error-free transmission. However, for links above 150 km the performance deteriorates to such an extent that the signal becomes undetectable.
In-Line Amplifier
These amplifiers are used for compensating distribution losses in local-area networks. replace electronic regenerators. An in-line amplifier is characterized by large gain and low noise to amplify an already attenuated signal so that it can travel an additional length of fiber.
In-line amplifiers are placed every 80-100 km to ensure that the optical signal level remains above the noise floor. In-line amplifiers typically require moderate gain in the range of 15-25 dB, and NF in the range of 5-7 dB. Output power requirements are similar to those of booster amplifiers. While in the early days of optical amplifiers different amplifier models had to be specifically tailored for each of the above functions, today the technology has advanced so that a single well designed amplifier model can perform many of the functions for typical applications. However, there still remain challenging applications which require specially designed amplifiers, such as very high output power boosters, or ultra-low noise pre-amplifiers.
Question 14: What is power control mode and Gain control mode?
Power Control Mode:
When amplifier works in power control mode, it maintains constant per channel power when desired or accidental changes to the number of channels occur. Constant per channel power increases optical network resilience.
Gain Control mode:
In constant gain mode, the amplifier power out control loop performs the following input and output power calculations, where G represents the gain and t represents time.
Pout (t) = G * Pin (t) (mW)
Pout (t) = G + Pin (t) (dB)
Question 15: What is the effect of Amplifier gain on OSNR while reducing or increasing flat gain?
A common way to characterize the performance of an amplifier is through its Noise figure. The noise figure is defined as the ratio of the signal to noise ratio at
the input of the amplifier-receiver to that at the output of the amplifier:
NF= SNR in/SNR out
The noise figure will always be greater than one, due to the fact that the amplifier Adds noise during the amplification process and the signal to noise ratio at the output is always lower than that at the input. The noise figure value is usually given in dB.
Noise output power ASE (Amplified Spontaneous Emission) directly depends on Gain of Amplifier. The OSNR at any point in a fiber link is equal to the signal power divided by the noise power. If Noise power will increase OSNR will decrease and will introduce errors in channel.
But in link where multiple EDFA deployed, gain is set as per planning, but there is always some margin, for gain adjustment. If you will increase Gain of first few EDFA, as this gain will increase noise power (ASE) and this ASE will mix with Signal power and with signal power, noise power will be amplified by downstream EDFA, and this ASE will degrade final OSNR of channels.
Question 16: What is gain tilt and Gain ripple?
The ability to control and adjust per channel optical power equalization is a principal feature of Amplifier in network applications. A major parameter to assure optical spectrum equalization throughout the DWDM system is the gain flatness of erbium-doped fiber amplifiers (EDFAs).
Effect of Gain Ripple and Gain Tilt on Amplifier Output Power are as follows:
Gain ripple:
Gain ripple is random and it depends on the spectral shape of the amplifier optical components.
Gain tilt:
Gain tilt is systematic and it depends on the gain set point of the optical amplifier, which is a mathematical function that relates to the internal design of the amplifier.
Gain tilt is the only contribution to the power spectrum dis-equalization that can be compensated at the module level. A VOA inside the amplifier can be used to compensate for gain tilt. An optical spectrum analyzer (OSA) is used to acquire the output power spectrum of an amplifier. The OSA shows the peak-to-peak difference between the maximum and minimum power levels, and takes into account the contributions of both gain tilt and gain ripple.
Question 17: What is a 30 dB gain means?
30dB gain means for every input PHOTON there will be 1000 PHOTON’s at output. That’s what Gain is.
X dB is 10x/10
Question 18: What is Raman Amplifier and how does it work?
Raman amplifier is a well-known amplifier configuration. This amplifier uses conventional fiber (rather doped fibers), which may be co-or counter-pumped to provide amplification over a wavelength range which is a function of the pump wavelength. The Raman amplifier relies upon forward or backward stimulated Raman scattering. Typically, the pump source is selected to have a wavelength of around 100 nm below the wavelength over which amplification is required.
Principle of working:
As the pump laser photons propagate in the fiber, they collide and are absorbed by fiber molecules or atoms. This excites the molecules or atoms to higher energy levels. The higher energy levels are not stable states so they quickly decay to lower intermediate energy levels releasing energy as photons in any direction at lower frequencies. This is known as spontaneous Raman scattering or Stokes scattering and contributes to noise in the fiber.
Since the molecules decay to an intermediate energy vibration level, the change in energy is less than the initial received energy during molecule excitation. This change in energy from excited level to intermediate level determines the photon frequency since Δ f = Δ E / h. This is referred to as the Stokes frequency shift and determines the Raman gain versus frequency curve shape and location. The remaining energy from the intermediate level to ground level is dissipated as molecular vibrations (phonons) in the fiber. Since there exists a wide range of higher energy levels, the gain curve has a broad spectral width of approximately 30 THz.
During stimulated Raman scattering, signal photons co-propagate frequency gain curve spectrum, and acquire energy from the Stokes wave, resulting in signal amplification.
Some of the information bullet to know is:
• The Raman amplifier is typically much more costly and has less gain than an Erbium Doped Fiber Amplifier (EDFA) amplifier. Therefore, it is used only for specialty applications.
• The main advantage that this amplifier has over the EDFA is that it generates very less noise and hence does not degrade span Optical to Signal Noise Ratio (OSNR) as much as the EDFA.
• Its typical application is in EDFA spans where additional gain is required but the OSNR limit has been reached.
• Adding a Raman amplifier might not significantly affect OSNR, but can provide up to a 20dB signal gain.
• Another key attribute is the potential to amplify any fiber band, not just the C band as is the case for the EDFA. This allows for Raman amplifiers to boost signals in O, E, and S bands (for Coarse Wavelength Division Multiplexing (CWDM) amplification application).
• The amplifier works on the principle of Stimulated Raman Scattering (SRS), which is a nonlinear effect.
• It consists of a high-power pump laser and fiber coupler (optical circulator).
• The amplification medium is the span fiber in a Distributed Type Raman Amplifier (DRA).
• Raman amplifiers can work at any wavelength as long as the pump wavelength is suitably chosen. It can work in C and L bands.
• Distributed Feedback (DFB) laser is a narrow spectral bandwidth which is used as a safety mechanism for Raman Card. DFB sends pulse to check any back reflection that exists in the length of fiber. If no High Back Reflection (HBR) is found, Raman starts to transmit.
• Generally, HBR is checked in initial few kilometers of fibers to first 20 Km. If HBR is detected, Raman will not work. Some fiber activity is needed after you find the problem area via OTDR.
Question 19: What are the advantages of using Raman Amplifier?
• Its usage improves the overall gain characteristics of high capacity optical wavelength division multiplexed (WDM) communications systems.
• Its usage do not attenuate signals outside the wavelength range over which amplification takes place.
• Raman amplifiers can work at any wavelength as long as the pump wavelength is suitably chosen. This property, coupled with their wide bandwidth, makes Raman amplifiers quite suitable for WDM systems.
Question 20: What are the Noise sources in Raman Amplifier?
Major Noise sources of Raman Amplifiers are:
▪ Amplified spontaneous emissions (ASE)
▪ Double Rayleigh scattering (DRS)
▪ Pump laser noise.
ASE noise is due to photon generation by spontaneous Raman scattering.DRS noise occurs when twice reflected signal power due to Rayleigh scattering is amplified and interferes with the original signal as crosstalk noise. The strongest reflections occur from connectors and bad splices. Typically, DRS noise is less than ASE noise, but for multiple Raman spans it can add up. To reduce this interference, ultra-polish connectors (UPC) or angle polish (APC) connectors can be used. Optical isolators can be installed after the laser diodes to reduce reflections into the laser. Also span OTDR traces can help locate high-reflective events for repair.
Counter pump DRA configuration results in better OSNR performance for signal gains of 15 dB and greater. Pump laser noise is less of a concern because it usually is quite low with RIN of better than 160 dB/Hz.
Nonlinear Kerr effects can also contribute to noise due to the high laser pump power. For fibers with low DRS noise, the Raman noise figure due to ASE is much better than the EDFA noise figure. Typically, the Raman noise figure is –2 to 0 dB, which is about 6 dB better than the EDFA noise figure.
Raman amplifier noise factor is defined as the OSNR at the input of the amplifier to the OSNR at the output of the amplifier.
An undesirable feature is that the Raman gain is somewhat polarization sensitive. In general, the gain is maximum when the signal and pump are polarized along the same direction but is reduced when they are orthogonally polarized.
Question 21: What is the significance of forward pumping and backward pumping Raman amplifiers in a link?
Forward pumping provides the highest SNR, and the smallest noise figure, because most of the Raman gain is then concentrated toward the input end of the fiber where power levels are high. However, backward pumping is often employed in practice because of other considerations such as the transfer of pump noise to signal and the effects of residual fiber birefringence.
Question 22: Why generally EDFA and Raman are used in conjunction to each other?
Counter pump distributed Raman amplifiers are often combined with EDFA pre-amps to extend span distances. This hybrid configuration can provide 6 dB improvement in the OSNR, which can significantly extend span lengths or increase span loss budget. Counter pump Raman Amplifier can also help reduce nonlinear effects by allowing for channel launch power reduction.
Raman Amplifiers are very sensitive to input power so they are always used with EDFA in cascaded fashion. (a small change at input will result in high output power change and thus subsequent components may suffer)
Question 23: What are linear effects?
Each data channel in a DWDM link is a train of pulses. Being finite in time, each optical pulse is composed of a range of wavelengths distributed around a central optical wavelength, which corresponds to the central wavelength of a specific DWDM channel. The total signal in the optical fiber is then the combination of all the DWDM optical channels multiplexed in the optical fiber. During propagation in the optical fiber, the shape and amplitude of each pulse is modified by various effects arising from the physical properties of the optical fiber material.
Linear Impairments: Impairments increases linearly as signal propagates in fiber with distance known as linear impairments like attenuation and dispersion.
Question 24: What are nonlinear effects?
Nonlinear effects are the impairments in optical signal caused by interaction of power levels of various signals in fiber. Non-linear interactions between the signal and the silica fiber transmission medium begin to appear as optical signal powers are increased to achieve longer span lengths at high bit rates. Consequently, non-linear fiber behavior has emerged as an important consideration both in high capacity systems and in long unregenerated routes.
A variety of parameters influence the severity of these non-linear effects, including line code (modulation format), transmission rate, fiber dispersion characteristics, the effective area and non-linear refractive index of the fiber, the number and spacing of channels in multiple channel systems, overall unregenerated system length, as well as signal intensity and source line-width.
Question 25: What are the types of Nonlinear effects that happens in a DWDM link?
These nonlinear interactions can be divided into three main categories:
(1) Brillouin effect,
(2) Kerr effect, and
(3) Raman effect.
Stimulated Brillouin Scattering (Brillouin effect)
Stimulated Brillouin scattering (SBS) is an inelastic phenomenon resulting from the scattering of photon inside the optical fiber. The scattered photon is slightly frequency downshifted compared to the initial photon, the energy difference being transferred to an acoustic phonon.
When increasing the launch power, the optical fiber practically acts as a mirror whose reflectance coefficient increases. As a result, the corresponding fiber loss can significantly grow and the induced reflections can degrade the system performance.
When low power is injected into the fiber, only intrinsic Rayleigh back-reflections occur and the level of reflections is very low (around 32 dB). When high power is launched in the fiber, the backscattered power increases because of the stimulated Brillouin scattering
The SBS-related penalty can be minimized by keeping the per-channel power below the SBS threshold, which depends on the size of the optical fiber core and on the transmitter linewidth
Kerr Effect
In the case of a single-channel transmission, the refractive index of the waveguide is modulated by the fluctuations of the channel intensity via the Kerr effect. The amplitude of this phenomenon is increased by a high launch power and small effective area inside the optical fiber. This nonlinear effect can broaden the channel spectrum and therefore interplay with the chromatic dispersion, resulting in pulse distortion and broadening.
The Kerr effect is usually decomposed in three different contributions that are actually closely related. When a signal travels alone through the fiber, its modulated power induces a self-phase modulation (SPM). By contrast, the presence of several channels in a WDM transmission generates on each signal a cross-phase modulation. For the particular case of well-phase-matched WDM signals (i.e. moderate fiber chromatic dispersion), the Kerr effect produces four- wave mixing (FWM).
1. Self-Phase Modulation
Light travels more slowly when the optical power is high, leading to a phase difference compared to light traveling at a low optical power. The result of the propagation of an amplitude-modulated signal is known as SPM.Self-phase modulation becomes significant as soon as the launch power is typically larger than 12 dBm.
2. Cross-Phase Modulation
In the case of several high-power channels propagating simultaneously within the same fiber, the refractive index modulation experienced by one given channel is not only caused by the intensity modulation of this specific channel (SPM) but also by the intensity modulation brought by the copropagating channels. This cross-refractive index modulation is called cross-phase modulation (XPM) and can be described as a process through which the intensity fluctuations in a particular channel are converted to phase fluctuations in the other channels.
3. Four-Wave Mixing
When several carriers at different wavelengths are launched into the fiber and are closed to be phase-matched, new waves can be generated by four-wave mixing via third-order intermodulation process. The optical frequencies of these FWM-generated waves are given by nijk= ni
+ nj -nk where ni ; nj , and nk are the frequencies of the launched initial channels (i.e., the signal channels). Four-wave mixing can transfer a fraction of the channel powers to the frequency of the other channels through the generation of FWM waves. FWM is considered the most dominant source of crosstalk in WDM systems .It becomes a major source of nonlinear crosstalk when- ever the channel spacing and fiber dispersion are small enough to satisfy the phase- matching condition approximately. For an N-channel system, i, j , and k can vary from 1 to N, resulting in a large combination of new frequencies generated by FWM. In the case of equally spaced channels, the new frequencies coincide with the existing frequencies, leading to coherent in-band crosstalk. When channels are not equally spaced, most FWM components fall in between the channels and lead to incoherent out-of-band crosstalk. In both cases, system performance is degraded because power transferred to each chan- nel through FWM acts as a noise source, but the coherent crosstalk degrades system performance much more severely.
Stimulated Raman Scattering
Like SBS, stimulated Raman scattering (SRS) is an inelastic phenomenon resulting from the scattering of an incoming photon inside the optical fiber. The scattered photon is frequency downshifted compared to the initial photon, the energy difference being transferred to an optical phonon. When several beams propagate through the fiber at different wavelengths, the maximum energy transfer occurs for a 13.2-THz separation between the channels .
Channel interaction due to Raman scattering is not maximal for channel spacing lower than 13.2 THz, which is the case for WDM systems; nevertheless, it can still be significant for high-power, wideband systems.
Question 26: What is impact of linear and nonlinear effects in DWDM network?
Work of transmission systems is to transmit signal from one location to another location over media and receive signal error free. Lot of impairments caused by Transmission media, system components and by signal itself. As in DWDM network each channel which carries signals like SDH, SONET, and Ethernet propagates in optical domain it encounters with linear and nonlinear effects, which distort signal pulse shape and amplitude. As each signal generated and received by Transceivers or Transponders. When this distorted optical signal received by Transceiver, it converts the optical signal in electrical signal, which decodes the actual information like SDH, SONET or Ethernet carry over individual channel. In digital format the impact of optical Linear and nonlinear impairments are detected as BER. Because these impairments, changed the original patterns of pulse shape and amplitude of signal, pulse width expansion leads to inter symbol interference, and at receiver end this is decoded 1 as 0 or 0 as 1, which cause BER.
Question 27: How to reduce FWM impact?
One method to reduce FWM effects is to use transmission fiber that has high chromatic dispersion coefficient at the signal wavelength such as standard single-mode fiber SSMF (ITU-T G.652). The typical chromatic dispersion coefficient of SSMF is 18 ps/nm ⋅ km @ 1550 nm which helps to significantly reduce FWM from occurring. Even a lower chromatic dispersion coefficient significantly helps in reducing FWM such as NZ-DSF fiber (ITU-T G.655). This fiber is specially designed with low chromatic dispersion coefficient (~4 ps/nm ⋅ km @ 1550 nm) for extended transmission distance but high enough to significantly reduce FWM effects.
To reduce these effects is to lower signal power in the fiber or use fiber with a larger cross sectional area to reduce the signal power density.
A third method to help reduce FWM effects is to space signal channels unevenly.
A fourth method to reduce FWM effects is to use DWDM systems with wide channel spacing. The magnitude of FWM effect is dependent on channel spacing. Wider spaced
DWDM channels generate weaker FWM components.
Question 28: How to know if errors are due to linear or non-linear issue in an optical link during troubleshooting errors?
When the bit error occurs to the system, generally the OSNR at the transmit end is well and the fault is well hidden.
Decrease the optical power at the transmit end at that time. If the number of bit errors decreases at the transmit end, the problem is non-linear problem.
If the number of bit errors increases at the transmit end, the problem is the OSNR degrade problem.
Question 29: What are different types of fibers and what is their significance?
Significance | ITU-T Standards | Characteristics | Wavelength Coverage | Applications |
50/125µm Graded-Index Multimode Fiber for FTTH Systems | G.651.1 | Cladding Diameter & Core Diameter:125 ±2 µm; 50 ±3 µmMacrobend loss: 15mmAttenuation:”Max at 850 nm: 1 dBMax at 1300 nm: 1 dBMax at 850 nm: 3.5 dB/kmMax at 1300 nm: 1.0 dB/km” | 850 nm;1300 nm | Support FTTH and FTTZ architectures; Recommend the use of quartz multimode fiber for access networks in specific environments. |
Standard Single-Mode Fiber for CWDM Systems | G.652.A | Max PMDQ=0.5 ps/√ km | O and C bands | Support applications such as those recommended in ITU-T G.957 and G.691 up to STM-16, as well as 10 Gbit/s up to 40 km(Ethernet) and STM-256 for ITU-T G.693. |
G.652.B | Maximum attenuation specified at 1625 nm.Max PMDQ=0.2 ps/√ km | O, C and L bands | Support higher bit-rate applications up to STM-64, such as some in ITU-T G.691 and G.692, and STM-256 for applications in ITU-T G.693 and G.959.1. | |
G.652.C | Maximum attenuation specified at 1383 nm (equal or lower than 1310 nm).Max PMDQ=0.5 ps/√ km | O, E, S, C and L bands | Similar to G.652.A, but this standard allows transmission in portions of an extended wavelength range from 1360 nm to 1530 nm. Suitable for CWDM systems. | |
G.652.D | Maximum attenuation specified from 1310 to 1625 nm. Maximum attenuation specified at 1383 nm (equal or lower than 1310 nm).Max PMDQ=0.2 ps/√ km | O, E, S, C and L bands | Similar to G.652.B, but this standard allows transmission in portions of an extended wavelength range from 1360 nm to 1530 nm. Suitable for CWDM systems. | |
Dispersion-Shifted Single-mode Optical Fiber for Long Haul Transmission | G.653.A | Zero chromatic dispersion value at 1550 nm. Maximum attenuation of 0.35 dB/km at 1550 nm. Max PMDQ=0.5 ps/√ km | 1550 nm | Supports high bit rate applications at 1550 nm over long distances. |
G.653.B | Maximum attenuation specified at 1550 nm only. Max PMDQ=0.2 ps/√ km | 1550 nm | With a low PMD coefficient, this standard supports higher bit rate transmission applications than G.653.A. | |
Cut-off Shifted Single-mode Fiber for Long Haul Submarine & Terrestrial Networks | G.654.A | Maximum attenuation of 0.22 dB/km at 1550 nm.Max PMDQ=0.5 ps/√ km | 1550 nm | Suited for long-distance digital transmission applications, such as long-haul terrestrial line systems and submarine cable systems using an optical amplifier. |
G.654.B | Maximum attenuation of 0.22 dB/km at 1550 nm.Max PMDQ=0.20 ps/√ km | 1550 nm | Same ITU-T system as G.654.A and for ITU-T G.69.1 long-haul applications in the 1550 nm region. Also suited for longer distance and larger WDM repeaterless submarine systems with remotely pumped optical amplifiers in G.973. Also, for submarine systems with optical amplifiers in G.977 | |
G.654.C | Maximum attenuation of 0.22 dB/km at 1550 nm.Max PMDQ=0.20 ps/√ km | 1550 nm | Suited for higher bit-rate and long-haul applications in G.959.1. | |
G.654.D | Maximum attenuation of 0.20 dB/km at 1550 nm.Max PMDQ=0.20 ps/√ km | 1550 nm | Suited for higher bit-rate submarine systems in G.973, G.973.1, G.973.2, and G.977. | |
G.654.E | Maximum attenuation of 0.23dB/km at 1550nm.Max PMDQ=0.20 ps/√ km | 1550 nm | Similar to ITU-T G.654.B, but has a smaller macrobending loss specification equivalent to ITU-T G.652.D fibers, and a tightened range of nominal MFD. For deployment as terrestrial cables with improved OSNR characteristics to support higher bit-rate coherent transmission, e.g., 100G/200G/400G systems. | |
Legacy Long Haul Single-mode Fiber for CWDM System | G.655.A | Maximum attenuation at 1550 nm only. Lower CD value than B and C category. Max | C band | Support DWDM transmission (G.692) applications in the C band with down to 200GHz channel spacing. |
G.655.B | Maximum attenuation specified at 1550 and 1625 nm. Max PMDQ=0.5 ps/√ km | C+L band | Support DWDM transmission (G.692) applications in the C+L band with down to 100GHz channel spacing. | |
G.655.C | Maximum attenuation specified at 1550 and 1625 nm. Max PMDQ=0.2 ps/√ km | O to C band | Similar to G.655.B, but allows for transmission applications at high bit rates for STM-64 (10 Gbps) up to 2000 km. Also suitable for STM-256 (40 Gbps). | |
G.655.D | Maximum attenuation specified at 1550 and 1625 nm. Max PMDQ=0.2 ps/√ km | C+L band | For wavelengths greater than 1530 nm. Similar applications to G.655.B are supported. For wavelength, less than 1530 nm, can support CWDM applications at channels 1471 nm and higher. | |
G.655.E | Maximum attenuation specified at 1550 and 1625 nm. Max PMDQ=0.2 ps/√ km | C+L band | Similar to G.655.D, but have higher CD values for applications with small channel spacing. | |
Non-zero Dispersion Fiber for CWDM and DWDM System | G.656 | Maximum attenuation at 1460, 1550, and 1625 nm. Max PMDQ=0.2 ps/√ km | S, C and L band | Supports both CWDM and DWDM systems throughout the wavelength range of 1460 nm to 1625 nm. |
Bend-insensitive Single-mode Fiber for FTTH Systems | G.657.A | At 15 mm radius, 10 turns, 0.25 dB max at 1550 nm, 1 dB max at 1625 nm. Max PMDQ=0.20 ps/√ km | from O to L band | Optimized access installation with respect to macro bending, loss, other parameters being similar to G.652.D. |
G.657.B | At 15 mm radius, 10 turns, 0.03 dB max at 1550 nm, 0.1 dB max at 1625 nm | from O to L band | Supports optimized access network installation with very small bending radii applied in fiber management systems and particularly for restricted distance installations. |
Question 30: What are Fiber spectrum bands?
Band Description | Wavelength Range (nm) |
850 Multimode Window | 800-910 |
O Original | 1260-1360 |
E Extended | 1360-1460 |
S Short | 1460-1530 |
C Conventional (EDFA Window) | 1530-1565 |
L Long (Extended EDFA) | 1565-1625 |
U Ultra Long Haul | 1625-1675 |
Question 31: What is Red and Blue and Red Band is preferred over Blue in DWDM?
The ITU approved DWDM band extends from 1528.77 nm to 1563.86 nm, and divides into the red band and the blue band.
The red band encompasses the longer wavelengths of 1546.12 nm and higher.
The blue band wavelengths fall below 1546.12 nm.
This division has a practical value because useful gain region of the lowest cast EDFAs corresponds to the red band wavelengths. Thus, if a system only requires a limited number of DWDM wavelengths using the red band wavelength yields the lowest overall system cost.
Regarding Red and Blue convention.
It’s just a convention which is prevalent since electromagnetic spectrum is in study either it is Doppler effect or Rayleigh Scattering and later on it was taken into consideration in optics or photonics world.
(Taken from Wikipedia:)
It’s more of talking light spectrum VIBGYOR where red-shift and blue-shift is discussed and “red-shift “ happens when light or other electromagnetic radiation from an object is increased in wavelength, or shifted to the red end of the spectrum.
In general, whether or not the radiation is within the visible spectrum, “redder” means an increase in wavelength – equivalent to a lower frequency and a lower photon energy,
A blueshift is any decrease in wavelength, with a corresponding increase in frequency, of an electromagnetic wave; the opposite effect is referred to as redshift. In visible light, this shifts the color from the red end of the spectrum to the blue end.
The ITU approved DWDM C-band extends from 1528.77 nm to 1563.86 nm, and divides into the red band and the blue band.
The red band encompasses the longer wavelengths of 1546.12 nm and higher.
The blue band wavelengths fall below 1546.12 nm.
Example to make it clearer:-
C Band: 1528.77 nm to 1563.86 nm
C-Blue 1529.44~1543.84
=====guard band====
C-red 1547.60~1561.53
L Band: 1565nm-1625nm
L-Blue: 1570nm-1584nm
=====guardband====
L-Red: 1589nm-1603nm
So, this blue and red shift is for characterization behavior study and to classify filters as well .
Question 32: What is dark fiber, dim fiber and lit fiber?
The term “dark fiber” refers to optical fibers that are not connected to any light wave equipment and hence they are dark. Fiber optic cables often contain a great many fibers, some of which are lit, and others of which are left unlit, or dark. The dark fibers can be spares for backup purposes, can be held in reserve to accommodate future demand, or can be available for lease or sale to other carriers or user organizations with private line requirements. Dark fibers are also used to study characterization of fiber since it is not carrying any services.
A dim fiber is one over which not all available wavelengths have been lit and which, therefore, has excess capacity.
Lit Fiber is Optical fiber that is regularly being used to transmit data
Question 33: What are the sources of latency in optical fiber?
Latency is a time delay experienced in system and it describes how long it takes for data to get from transmission side to receiver side. In a fiber optical communication system, it is essentially the length of optical fiber divided by the speed of light in fiber core, supplemented with delay induced by optical and electro optical elements plus any extra processing time required by system, also called overhead. Signal processing delay can be reduced by using parallel processing based on large scale integration CMOS technologies.
Added to the latency due to propagation in the fiber, there are other path building blocks that affect the total data transport time. These elements include
▪ opto-electrical conversion,
▪ switching and routing,
▪ signal regeneration,
▪ Amplification,
▪ chromatic dispersion (CD) compensation,
▪ polarization mode dispersion (PMD) compensation,
▪ data packing, digital signal processing (DSP),
▪ protocols and addition forward error correction (FEC)
Question 34: What is micro bending and macro bending?
Macro bending: Macrobending is the attenuation associated with bending or wrapping the fiber. Light can “leak out” of a fiber when it is bent. As the bend becomes tighter, more light escapes. Macrobending loss, measured in decibels, increases at longer wavelengths where the optical confinement of the light is weaker. It also increases linearly with the number of turns. Traditionally, macrobending was not a limiting effect when cables were mostly of loose-tube or ribbon design and installed into ducts. The tightest bends incurred by fibers were in splice trays, where excess fiber would be stored in loops after jointing. This was reflected in the macrobending specification of ITU-T Recommendation G.652, where a minimum bend radius of 30 mm was defined to reflect typical splice tray dimensions and 100 turns were agreed upon to simulate the total excess fiber from all the splice sites between repeaters. But macrobending effects become more pronounced in networks installed close-to and within the building. Prevalent in this segment of the network are low-diameter mini-cables that are stripped-back designs, compared to the traditional sheathed loose-tube and ribbon cables. Lightweight and highly flexible, these new designs are preferred for their space efficiency (when installed into commensurately small micro-ducts) and ease of handling and routing (when installed on the inside and outside of buildings along tortuous paths). Bend radii of much less than 30 mm therefore have become commonplace.
Micro bending :Microbending attenuation of an optical fiber relates to the light signal loss associated with lateral stresses along the length of the fiber. The loss is due to the coupling from the fiber’s guided fundamental mode to lossy, higher-order radiation modes. Mode coupling occurs when fibers suffer small random bends along
the fiber axes. This random bending is usually caused by external mechanical stresses against the cable material that compress the fiber. The result is random, high-frequency perturbations to the fiber. Lateral stresses can be caused by pressure induced by manufacturing or installation or by temperature-induced dimensional changes in cabling materials that cause undesirable fiber/fiber or fiber/cable material interactions. These interactions can give rise to random microscopic bends or curvatures of <1-mm radius that create very small displacements of the fiber core from the fiber axis. Microbending effects can be seen at all the commonly used wavelengths in single mode fibers (1310, 1550, and 1625 nm), whereas macrobending effects are seen predominantly at 1550 and 1625 nm.
Question 35: What is fiber characterization?
Fiber characterization can be defined as the field measurement and recording of fiber span parameters that affect signal transmission over all or selected operating wavelengths. These measured parameters provide a true picture of the fiber span’s transmission limitations. They are used in network planning to ensure transmission links are designed within transceiver operating budgets and limits. Full fiber characterization is often necessary in modern high-speed link designs, where optical budgets are stretched to their maximum with little or no margin for error. Fiber quality can also be assessed with these parameters. Fiber characterization is performed after new fiber cable link construction, dark fiber purchase, or lease. This helps to ensure the fiber quality meets or exceeds required specifications and expectations. It also documents fiber parameters at the time of construction or acquisition for comparison with future measurements to determine fiber degradation due to aging, damage, and repair.
Question 36: What is PMD?
Polarization mode dispersion (PMD) is a property of a single-mode fiber or an optical component where pulse spreading is caused by different propagation velocities of the signal’s two orthogonal polarizations. Optical fibers or optical components can be modeled with two orthogonal polarization axes called principal states of polarization (PSP).
An optical signal propagating in a fiber is resolved into these two PSP axes. Each polarization axis (fast and slow axis) has a different propagation velocity. This is due to different refractive indexes in each axis caused by the birefringence of the material. The different velocities lead to pulse spreading at the receiver end.
Question 37: What is PMD coefficient and its unit?
PMD can be expressed as the square root of the fiber length multiplied by a proportionality coefficient. This coefficient is referred to as the PMD coefficient and is measured in units of picoseconds per square root kilometer (ps/√km). The PMD coefficient is typically specified by fiber cable manufacturers and represents the PMD characteristic for a particular length of that fiber.
Question 38: What is DGD?
The amount of pulse spreading in time between the two polarization pulses is referred to as differential group delay (DGD) and is measured in units of picoseconds. Note, the time it takes for a pulse to propagate in a fiber is referred to as the group delay. DGD is an instantaneous value that varies randomly along the length of a fiber.
Question 39: What is CD?
Chromatic dispersion (CD) is a property of optical fiber (or optical component) that causes different wavelengths of a light source to propagate at different velocities, means if transmitting signal, from a LASER source, this LASER source having spectral width and emit different wavelengths apart from its center wavelength. Since all light sources consist of a narrow spectrum of light (comprising of many wavelengths), all fiber transmissions are affected by chromatic dispersion to some degree. In addition, any signal modulating a light source results in its spectral broadening and hence exacerbating the chromatic dispersion effect. Since each wavelength of a signal pulse propagates in a fiber at a slightly different velocity, each wavelength arrives at the fiber end at a different time. This results in signal pulse spreading, which leads two inter-symbol Interference between pulses and increases bit errors
Question 40: What is cause of CD?
Chromatic dispersion is due to an inherent property of silica optical fiber. The speed of a light wave depends on the refractive index, n, of the medium within which it is traversing. In silica optical fiber, as well as many other materials, n changes as a function of wavelength. Thus, different wavelengths travel at slightly different speeds along the optical fiber. A wavelength pulse is composed of several wavelength components or spectra. Each of its spectral constituents travel at slightly different speeds within the optical fiber. The result is a spreading of the transmission pulse as it travels through the optical fiber.
Question 41: What is unit of CD and CD Coefficient?
The chromatic dispersion (CD) parameter is a measure of signal pulse spread in a fiber due to this effect. It is expressed with ps/nm units, where the picoseconds refer to the Signal pulse spread in time and the nanometers refer to the signal’s spectral width. Chromatic dispersion can also be expressed as fiber length multiplied by proportionality
Coefficient. This coefficient is referred to as the chromatic dispersion coefficient and is measured in units of picoseconds per nanometer times kilometer, ps/(nm ⋅ km). It is
Typically specified by the fiber the cable manufacturer and represents the chromatic dispersion characteristic for a 1 km length of fiber.
Question 42: Which are main factors for CD?
Chromatic dispersion affects all optical transmissions to some degree. These effects become more pronounced as the transmission rate increases and fiber length increases.
Factors contributing to increasing chromatic dispersion signal distortion include the following:
1. Laser spectral width, modulation method, and frequency chirp. Lasers with wider spectral widths and chirp have shorter dispersion limits. It is important to refer to manufacturer specifications to determine the total amount of dispersion that can be tolerated by the lightwave equipment.
2. The wavelength of the optical signal. Chromatic dispersion varies with wavelength in a fiber. In a standard non-dispersion shifted fiber (NDSF G.652), chromatic dispersion is near or at zero at 1310 nm. It increases positively with increasing wavelength and increases negatively for wavelengths less than 1310 nm.
3. The optical bit rate of the transmission laser. The higher the fiber bit rate, the greater the signal distortion effect.
4. The chromatic dispersion characteristics of fiber used in the link. Different types of fiber have different dispersion characteristics.
5. The total fiber link length, since the effect is cumulative along the length of the fiber.
6. Any other devices in the link that can change the link’s total chromatic dispersion including chromatic dispersion compensation modules.
7. Temperature changes of the fiber or fiber cable can cause small changes to chromatic dispersion. Refer to the manufacturer’s fiber cable specifications for values.
Question 43: How to mitigate CD in a link?
1. Change the equipment laser with a laser that has a specified longer dispersion limit. This is typically a laser with a narrower spectral width or a laser that has some form of pre-compensation. As laser spectral width decreases, chromatic dispersion limit increases.
2. For new construction, deploy NZ-DSF instead of SSMF fiber.NZ-DSF has a lower chromatic dispersion specification.
3. Insert chromatic dispersion compensation modules (DCM) into the fiber link to compensate for the excessive dispersion. The optical loss of the DCM must be added to the link optical loss budget and optical amplifiers may be required to compensate.
4. Deploy a 3R optical repeater (re-amplify, reshape, and retime the signal) once a link reaches chromatic dispersion equipment limit.
5. For long haul undersea fiber deployment, splicing in alternating lengths of dispersion compensating fiber can be considered.
6. To reduce chromatic dispersion variance due to temperature, buried cable is preferred over exposed aerial cable.
Question 44: What are general performance parameters available on an Optical Amplifiers?
· Operating wavelength range.
· Nominal input power range.
· Input range per channel.
· Nominal single wavelength input optical power.
· Nominal single wavelength output optical power.
· Noise figure.
· Nominal gain.
· Gain response time on adding dropping of channels.
· Channel gain.
· Gain flatness
· Input reflectance.
· Output reflectance.
· Maximum reflectance tolerance at input.
· Maximum reflectance tolerance at output.
· Multi-channel gain slope.
· Polarization dependent loss.
· Gain tilt
· Gain ripple.
Question 45: Is CD affecting Multi channel system (DWDM) or Single channel system (Like SDH, Ethernet links on fiber)?
Chromatic dispersion (CD) is a property of optical fiber (or optical component). So, it will affect all systems which are connected with fiber. CD is caused by fiber and optical components, while CD tolerance limit is specification of Transceiver (SFP, Transponder).
Question 46: Which are factors contributing in DWDM design to increasing chromatic dispersion signal distortion?
Following are factors contributing in DWDM design to increasing chromatic dispersion signal distortion
1. Laser spectral width, modulation method, and frequency chirp. Lasers with wider spectral widths and chirp have shorter dispersion limits. It is important to refer to manufacturer specifications to determine the total amount of dispersion that can be tolerated by the light wave equipment.
2. The wavelength of the optical signal. Chromatic dispersion varies with wavelength in a fiber. In a standard non-dispersion shifted fiber (NDSF G.652), chromatic dispersion is near or at zero at 1310 nm. It increases positively with increasing wavelength and increases negatively for wavelengths less than 1310 nm.
3. The optical bit rate of the transmission laser. The higher the fiber bit rate, the greater the signal distortion effect.
4. The chromatic dispersion characteristics of fiber used in the link. Different types of fiber have different dispersion characteristics,
5. The total fiber link length, since the effect is cumulative along the length of the fiber.
6. Any other devices in the link that can change the link’s total chromatic dispersion including chromatic dispersion compensation modules.
7. Temperature changes of the fiber or fiber cable can cause small changes to chromatic dispersion. Refer to the manufacturer’ fiber cable specifications for values.
Question 47: What are methods to reduce CD in link?
Methods to reduce link chromatic dispersion are as follows:
1. Change the equipment laser with a laser that has a specified longer dispersion limit. This is typically a laser with a narrower spectral width or a laser that has some form of pre compensation. As laser spectral width decreases, chromatic dispersion limit increases.
2. For new construction, deploy NZ-DSF instead of SSMF fiber. NZ-DSF has a lower chromatic dispersion specification.
3. Insert chromatic dispersion compensation modules (DCM) into the fiber link to compensate for the excessive dispersion. The optical loss of the DCM must be added to the link optical loss budget and optical amplifiers may be required to compensate.
4. Deploy a 3R optical repeater (re-amplify, reshape, and retime the signal) once a link reaches chromatic dispersion equipment limit.
5. For long haul undersea fiber deployment, splicing in alternating lengths of dispersion compensating fiber can be considered.
6. To reduce chromatic dispersion variance due to temperature, buried cable is preferred over exposed aerial cable.
Question 48: What is DCM or DSCM or DCU?
Chromatic dispersion compensation modules (DCM), also known as dispersion compensation units (DCU) or Dispersion slope compensation module (DSCM), can be added to an existing fiber link to compensate for high link dispersion totals. These DCM are made of various spool lengths of dispersion compensating fiber (DCF) or Fiber Bragg Grating (FBG) and provide fixed compensation. In DCF based DCM, their negative chromatic dispersion characteristics compensate for the transmission fiber’s positive dispersion, while in FBG due to grating for shorter signal wavelengths to be reflected sooner and have less propagation delay through the unit. Longer signal wavelengths travel further into the fiber grating before they are reflected and therefore have more propagation delay through the unit. This is the exact opposite of fiber chromatic dispersion and therefore helps reverse pulse spreading due to fiber dispersion. The length of the chirped fiber grating is typically between 10 and 100 cm.
The modules are typically specified by what length, in km, of standard G.652 fiber will be compensated or by the total dispersion compensation over a specific wavelength range, in ps/nm.
Question 49: What are considerations while deploying DCM?
DCM is typically deployed at the beginning or end of a fiber span to manage the chromatic dispersion. The following pointers should be considered when planning DCM deployment:
1. Do not exceed DCM maximum allowable input optical power.
2. Include the chromatic dispersion optical power penalty in optical budget plans.
3. Include DCM insertion loss in optical budget plans.
4. Optical amplifiers do not increase or decrease chromatic dispersion.
5. To minimize nonlinear distortion effects, maintain a small amount of residual dispersion in every span.
6. For 40 Gbps and higher systems, consider span pre-compensation to minimize intra channel nonlinear effects.
Question 50: What are the types of DCM in DWDM link?
Two types of DCM are used in the DWDM link and are called post-compensation and pre-compensation. Since DCMs are considered part of the transmission line, the prefixes “post-” (after) and “pre-” (before) refers to the section of the transmission line that requires the compensation.
For the post-compensation DCM deployment, DCMs are placed after the fiber span that needs compensation. For G.652 fiber compensation, dispersion remains positive throughout the link.
For the pre-compensation DCM deployment, DCMs are placed before the fiber span that needs compensation. For G.652 fiber compensation dispersion remains negative throughout the link.
Both methods are acceptable since optical amplifiers do not add dispersion into the link provided that the DCM maximum power specifications are not exceeded. Placing DCMs after the optical amplifier can reduce link OSNR, but may increase nonlinear distortions due to high power levels if DCMs use DCF fiber. DCF fiber is more susceptible to nonlinear effects due to its smaller core area. Optical amplifiers are available with intermediate stage access designed to accept DCM connections. This allows for
dispersion compensation with less impact on the link loss, OSNR, and nonlinear distortions.
Question 51: What is EDC?
Electronic Dispersion Compensation has been recognized as a technology that can mitigate power penalties associated with optical link budgets. The sources of the power penalty include inter-symbol interference (ISI) due to fiber chromatic and polarization mode dispersion, transmitter impairments, and non-ideal transmitter or receiver bandwidth (optic or electronic) limitations.
Electronic Dynamically Compensating Optics (eDCO) provides improved dispersion management and more extended reach. eDCO reduces the requirements of dispersion compensation in the DWDM network and allows channel agility.
Question 52: What is OSNR?
Some handy definition of OSNR to pick :
▪ OSNR [dB] is the measure of the ratio of signal power to noise power in an optical channel .

▪ OSNR is the short form of Optical Signal to Noise Ratio. It is key parameter to estimate performance of Optical Networks. It helps in BER calculation of Optical System.
▪ OSNR is important because it suggests a degree of impairment when the optical signal is carried by an optical transmission system that includes optical amplifiers.
▪ If we know the OSNR and the bandwidths, we can find Q and the BER
▪ It can be seen as the QoS at the physical layer of optical networks. OSNR is directly related to bit-error rate, which will lead to packet losses seen by higher layers.
▪ OSNR indirectly reflects BER and can provide a warning of potential BER deterioration.
▪ OSNR has long been recognized as a critical performance indicator for amplified high-speed transmission networks to ensure network performance and reliability, and it is related to many design parameters such as number or repeater/amplifiers, reach, available modulation formats, etc..
Now let’s explore it in more detail:
Optical signal-to-noise ratio (OSNR) is used to quantify the degree of optical noise interference on optical signals. It is the ratio of service signal power to noise power within a valid bandwidth. When the signal is amplified by the optical amplifier (OA), like EDFA, its optical signal to noise ratio (OSNR) is reduced, and this is the primary reason to have a limited number of OAs in a network.
The OSNR values that matter the most are at the receiver, because a low OSNR value means that the receiver will probably not detect or recover the signal. The OSNR limit is one of the key parameters that determine how far a wavelength can travel before regeneration.
OSNR serves as a benchmark indicator for the assessment of the performance of optical transmission systems. DWDM networks need to operate above their OSNR limit to ensure error-free operation. There exists a direct relationship between OSNR and bit error rate (BER), where BER is the ultimate value to measure the quality of a transmission.
The value of OSNR out that is needed to meet the required system BER depends on many factors such as the bit rate, whether and what type of FEC is employed, the magnitude of any crosstalk, or nonlinear penalties in the DWDM line segments, etc.
Optical amplifiers such as erbium-doped fiber amplifiers (EDFAs) are normally employed in optical networks to compensate for the transmission losses over long distances. However, besides providing optical gain, EDFAs also add unwanted amplified spontaneous emission (ASE) noise into the optical signal. Furthermore, the cascading of EDFAs results in the accumulation of ASE noise. ASE noise is typically quantified by OSNR and is one of the most critical parameters to be monitored in optical networks since the BER is directly related to the signal OSNR Furthermore, it also plays a pivotal role in fault diagnosis and as a measure of general health of links in an optical network.
Question 53: What is Optical Return Loss (ORL) in Optical Fiber system?
Few of the commonly used definition for ORL are:
1). When light passes through an optical component, most of it travels in the intended direction, but some light is reflected or scattered. In many applications, these reflections are unwanted because they can affect the emission characteristics of any laser in the system. In such applications, it is vital to measure the reflections for the components of the system. The Return Loss is defined as the light reflected into the input path. It is caused by scattering and reflection from optical surfaces like mirrors, lenses, and connectors or from defects, such as cracks and scratches. The back reflection is equal to the return loss with a negative quantity.
2). ORL is defined as the ratio (in dB) of the optical power (Pinc) traveling downstream at a system interface to the optical power reflected back upstream to the same interface. This includes the reflected power contributions from all system components downstream from the interface.
To clarify :
Reflectance (dB) = P reflected (dBm) – P incident(dBm)
A discrete reflection will always be a negative quantity as the reflected power cannot be greater than the incident power.
By convention, ORL is defined as:
ORL(dB) = P incident (dBm) – P reflected (dBm)
This means that ORL will always be a positive number. The fact that we want all power to move forward and none to be reflected means that the higher the positive number, the better.
3). The reflection factor for a component is a measure of how much light the component reflects. It is a ratio of the power reflected by the device to the power incident on the device. More normally, we talk about the return loss of a component. The return loss has units of dB. Return loss is given by:
Return Loss(dB) = –10log(Reflection Factor) (dB)
ORL(dB) = P incident (dBm) – P reflected (dBm)
4). Optical return loss is the ratio of the output power of the light source to the total amount of back-reflected power
(reflections and scattering). It is defined as a positive quantity.
ORL is measured in dB and is a positive value. Reflectance (dB) is the ratio of reflected power to incident power due to a single interface. It is defined as a negative quantity.
The higher the number, the smaller the reflection – yielding the desired result.
Question 54: What are the major sources of ORL?
System components such as
▪ connectors,
▪ mechanical splices,
▪ attenuators,
▪ patch cords
▪ glass/air terminations
All create a change in index of refraction as seen by an optical signal. The components are reflective in nature and can contribute to system ORL.
The fiber optic cable itself creates backscatter as light propagates through it. The amount of reflected power due to backscatter cannot be eliminated but is magnitudes smaller than the power from discrete reflections
Sources of loss include reflections and scattering along the fiber network. A typical Return Loss value for an Angled Physical Contact (APC) connector is about -55dB, while the RL from an open flat polish to air is typically about -14dB. High RL is a large concern in high bitrate digital or analog single mode systems and is also an indication of a potential failure point, or compromise, in any optical network.
Question 55: what are the implications of ORL?
The main effects of back-reflection due to ORL include the following:
▪ Less light is transmitted from the transmitter.
▪ Increase in light source interference
▪ Increasing the BER in digital transmission systems
▪ Multi path distortion can also occur.
▪ Reducing the OSNR in transmission
▪ Reflections can distort the optical signal as reflections travel back and forth between reflective components.
▪ Strong fluctuations in the laser output power.
▪ Increase in transmitter noise.
▪ Changes central wavelength and output power.
▪ Permanent damage to the laser.
Question 56: How does reflected power affect laser stability?
Reflected light can provide unwanted feedback to the laser cavity which will affect:
▪ Frequency Modulation Response changes
▪ Relative Intensity Noise (RIN)
▪ Optical frequency variations
▪ Laser line-width variations
Reflection induced degradation increases with system bit-rate !The end result is higher bit error rates (BER).
Question 57: How to test ORL?
The measurement of ORL is becoming more important in the characterization of optical networks as the use of wavelength-division multiplexing increases. These systems use lasers that have a lower tolerance for ORL, and introduce elements into the network that are located in close proximity to the laser.
The two major test methods:
Optical Continuous Wave Reflectometry (OCWR)
A laser source and a power meter, using the same test port, are connected to the fiber under test.
Optical Time Domain Reflectometry (OTDR)
The OTDR is able to measure not only the total ORL of the link but also section ORL.
Question 58: What are the methods to help improve ORL?
1. Use ultra-polish connectors do have low reflectance. So recommended to use APC connectors.
2.Fusion splices are recommended instead of mechanical connectors or mechanical splices where possible.
3. Perform fusion splice for point of reflection.
4.Install optical isolators at the laser to reduce back reflectance.
Typical Reflectance for few connectors:-
PC connecters: -30dB to –40 dB
UPC connectors: -40dB to –50dB
APC connectors : -60dB
Fiber to air interface on a PC connector: -14.7 dB
Rayleigh backscatter for telecom fiber: -70 dB/meter
The angle reduces the back-reflection of the connection.
Question 59: Why it is Good to have ORL >30dB for general fiber links?
Optical return loss (ORL) is the logarithmic ratio of the launch (incident) power divided by the total reflected power seen at the launch point. The total reflected power is the total accumulated reflected optical power measured at the launch caused by fiber Rayleigh scattering and Fresnel reflections. Rayleigh scattering is the scattering of light along the entire length of the fiber, caused by elastic collisions between the light wave and fiber molecules. This results in some of the light to be reflected to the source. Rayleigh scattering is intrinsic to the fiber and, therefore, cannot be eliminated.
Fresnel reflections occur in the light path where there is an abrupt change in the refractive index, such as at connections and splices. The further away a reflective event is from the fiber launch point, the less it contributes to the total reflected power. Therefore, fiber connections and splices closest to the laser contribute the most to the ORL. ORL is always expressed as a positive decibel. The higher the ORL, the lower the reflected power.
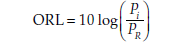
where ORL = optical return loss, dB
PR = total reflected power seen at the launch point, mW
Pi = launch or incident power, mW
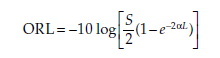
where S = backscattering capture coefficient, approximately 0.0015
for standard fiber at 1550 nm
L= fiber length, km
α= attenuation coefficient, 1/km
See calculation for ORL for SMF at 1550nm.
Assume fibre attenuation is 0.22 dB/km at 1550 nm,
S = 0.0015 with a nonreflective end.
L=20Km
After calculation using above generic values; ORL will come as ~30 dB.
ITU-T G.959.1 recommends a minimum ORL of 24 dB for 2.5, 10, and 40 Gbps fiber links.
Question 60: What is BER?
A Bit error rate, BER, is a key parameter that is used in assessing systems that transmit digital data from one location to another.
BER can be influenced by a number of factors. By manipulating the variables that can be controlled, it is possible to optimize a system to provide the performance levels that are required. This is normally undertaken in the design stages of a data transmission system so that the performance parameters can be adjusted at the initial design concept stages.
▪ Interference: The interference levels present in a system are generally set by external factors and cannot be changed by the system design. However, it is possible to set the bandwidth of the system. By reducing the bandwidth, the level of interference can be reduced. However, reducing the bandwidth limits the data throughput that can be achieved.
▪ Increase transmitter power: It is also possible to increase the power level of the system so that the power per bit is increased. This has to be balanced against factors including the interference levels to other users and the impact of increasing the power output on the size of the power amplifier and overall power consumption and battery life, etc.
▪ Lower order modulation: Lower order modulation schemes can be used, but this is at the expense of data throughput.
▪ Reduce bandwidth: Another approach that can be adopted to reduce the bit error rate is to reduce the bandwidth. Lower levels of noise will be received, and therefore the signal to noise ratio will improve. Again, this results in a reduction of the data throughput attainable.
It is necessary to balance all the available factors to achieve a satisfactory bit error rate. Normally it is not possible to achieve all the requirements, and some trade-offs are required. However, even with a bit error rate below what is ideally required, further trade-offs can be made in terms of the levels of error correction that are introduced into the data being transmitted. Although more redundant data has to be sent with higher levels of error correction, this can help mask the effects of any bit errors that occur, thereby improving the overall bit error rate.
Question 61: What is Q-factor and what is its importance?
Definition 1:
The Q-factor, a function of the OSNR, provides a qualitative description of the receiver performance. The Q-factor suggests the minimum signal-to-noise ratio (SNR) required to obtain a specific BER for a given signal. OSNR is measured in decibels. The higher the bit rate, the higher the OSNR ratio required. For OC-192 transmissions, the OSNR should be at least 27 to 31 dB compared to 18 to 21 dB for OC-48.
Definition 2:
The Quality factor is a measure of how noisy a pulse is for diagnostic purposes. The eye pattern oscilloscope will typically generate a report that shows what the Q factor number is. The Q factor is defined as shown in the figure: the difference of the mean values of the two signal levels (level for a “1” bit and level for a “0” bit) divided by the sum of the noise standard deviations at the two signal levels. A larger number in the result means that the pulse is relatively free from noise.
Definition 3:
Q is defined as follows: The ratio between the sums of the distance from the decision point within the eye (D) to each edge of the eye, and the sum of the RMS noise on each edge of the eye.
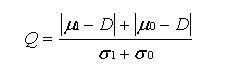
This definition can be derived from the following definition, which in turn comes from ITU-T G.976 (ref. 3).
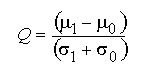
where m1,0 are the mean positions of each rail of the eye, and s1,0 are the S.D., or RMS noise, present on each of these rails.
For an illustration of where these values lie within the eye see the following figure:
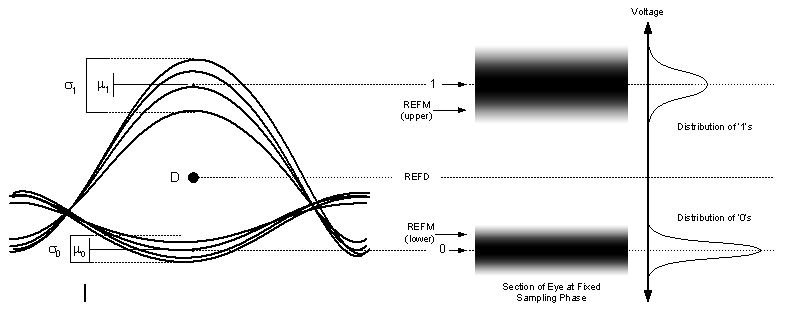
As Q is a ratio it is reported as a unit-less positive value greater than 1 (Q>1). A Q of 1 represents complete closure of the received optical eye. To give some idea of the associated raw BER a Q of 6 corresponds to a raw BER of 10-9.
Q FACTOR AS DEFINED IN ITU-T G.976
The Q factor is the signal-to-noise ratio at the decision circuit in voltage or current units, and is typically expressed by:
(A-1)
where µ1,0, is the mean value of the marks/space’s voltages or currents, and s1,0 is the standard deviation.
The mathematic relations to BER when the threshold is set to the optimum value are:
(A-2)
with:
(A-3)
The Q factor can be written in terms of decibels rather than in linear values:
(A-4)
CALCULATION OF Q-FACTOR FROM OSNR
The OSNR is the most important parameter that is associated with a given optical signal. It is a measurable (practical) quantity for a given network, and it can be calculated from the given system parameters. The following sections show you how to calculate OSNR. This section discusses the relationship of OSNR to the Q-factor.
The logarithmic value of Q (in dB) is related to the OSNR by following Equation
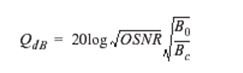
In the equation, B0 is the optical bandwidth of the end device (photodetector) and Bc is the electrical bandwidth of the receiver filter.
Therefore, Q(dB) is shown in
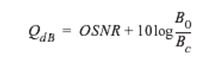
In other words, Q is somewhat proportional to the OSNR. Generally, noise calculations are performed by optical spectrum analyzers (OSAs) or sampling oscilloscopes, and these measurements are carried over a particular measuring range of Bm. Typically, Bmis approximately 0.1 nm or 12.5 GHz for a given OSA. From Equation showing Q in dB in terms of OSNR, it can be understood that if B0 < Bc, then OSNR (dB )> Q (dB). For practical designs OSNR(dB) > Q(dB), by at least 1–2 dB. Typically, while designing a high-bit rate system, the margin at the receiver is approximately 2 dB, such that Q is about 2 dB smaller than OSNR (dB).
The Qfactor, is in fact a metric to identify the attenuation in the receiving signal and determine a potential LOS and it-is an estimate of the Optical-Signal-to-Noise-Ratio (OSNR) at the optical receiver. As attenuation in the receiving signal increases, the dBQ value drops and vice-versa. Hence a drop in the dBQ value can mean that there is an increase in the Pre FEC BER, and a possible LOS could occur if the problem is not corrected in time.
Question 62: What are the advantages of Coherent Optical Transmission System?
• High Chromatic Dispersion (CD) Robustness
• Can avoid Dispersion Compensation Units (DCUs)
• No need to have precise Fiber Characterization
• Simpler Network Design
• Latency improvement due to no DCUs
• High Polarization Mode Dispersion (PMD) Robustness
• High Bit Rate Wavelengths deployable on all Fiber types
• No need for “fancy”PMD Compensator devices
• No need to have precise Fiber Characterization
• Low Optical Signal-to-Noise Ratio (OSNR) Needed
• More capacity at greater distances w/o OEO Regeneration
• Possibility to launch lower per-channel Power
• Higher tolerance to Channels Interferences
Question 63: Why Receiver Sensitivity is so important for optical module?
For Optical communication to happen, a receiver (essentially a photodetector, either a PIN or APD type) needs a minimum amount of power to distinguish the 0s and 1s from the raw input optical signal.
The minimum power requirement of the receiver is called the receiver sensitivity.
The optical power at the receiver end has to be within the dynamic range of the receiver;
otherwise, it damages the receiver (if it exceeds the maximum value) or the receiver cannot
differentiate between 1s and 0s if the power level is less than the minimum value.
Question 64: What is attenuation in Optical fiber?
Signals lose strength as they are travel through the fiber: this is known as attenuation. Attenuation is measured in decibels (dB) with the relation:
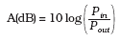
where Pin and Pout refer to the optical power going into and coming out of the fiber.
The attenuation of an optical fiber is wavelength dependent. At the extremes of the transmission curve, multi-photon absorption predominates. Attenuation is usually expressed in dB/km at a specific wavelength. Typical values range from 10 dB/km for step-index fibers at 850 nm to a few tenths of a dB/km for single-mode fibers at 1550 nm.
There are several causes of attenuation in an optical fiber:
Rayleigh Scattering — Microscopic-scale variations in the index of refraction of the core material can cause considerable scatter in the beam, leading to substantial losses of optical power. Rayleigh scattering is wavelength dependent and is less significant at longer wavelengths. This is the most important loss mechanism in modern optical fibers, generally accounting for up to 90 percent of any loss that is experienced.
Absorption — Current manufacturing methods have reduced absorption caused by impurities (most notably water in the fiber) to very low levels. Within the bandpass of transmission of the fiber, absorption losses are insignificant.
Bending — Manufacturing methods can produce minute bends in the fiber geometry. Sometimes these bends will be great enough to cause the light within the core to hit the core/cladding interface at less than the critical angle so that light is lost into the cladding material. This also can occur when the fiber is bent in a tight radius (less than, say, a few centimeters). Bend sensitivity is usually expressed in terms of dB/km loss for a particular bend radius and wavelength.
Question 65: What are the general colors of patch cord seen in Optical environment?
The buffer or jacket on patch cords are often color coded to indicate the type of fiber used. The strain relief “boot” that protects the fiber from bending at a connector is color-coded to indicate the type of connection. Connectors made with a plastic shell (such as SC connectors) typically use a color coded shell. Standard color coding’s for jackets and boots (or connector shells) are shown below:
Buffer/jacket color | Meaning |
Yellow | single-mode optical fiber |
Orange | multi-mode optical fiber |
Aqua | 10 gig laser-optimized 50/125 micrometer multi-mode optical fiber |
Grey | outdated color code for multi-mode optical fiber |
Blue | Sometimes used to designate polarization-maintaining optical fiber |
Connector Boot | Meaning | Comment | |
Blue | Physical Contact (PC), 0° | mostly used for single mode fibers; some manufacturers use this for polarization-maintaining optical fiber. | |
Green | Angle Polished (APC), 8° | ||
Black | Physical Contact (PC), 0° | ||
Grey, | Beige | Physical Contact (PC), 0° | multimode fiber connectors |
White | Physical Contact (PC), 0° | ||
Red | High optical power. Sometimes used to connect external pump lasers or Raman pumps. | ||
Question 66: Defining Colorless, Directionless, Contention-less flexible grid Network?
CDC allows operators to future proof their network, so they are able to optimize, scale, and flexibly meet any future bandwidth demands.
▪ Directionless: for the ability to route a wavelength across any viable path in the network
▪ Colorless: for the ability to receive any wavelength on any port.
▪Contentionless: eliminates wavelength blocking, allowing the add/drop of a duplicate wavelength onto a single mux/demux
▪ Flexible grid: for the ability to future-proof the network for any higher capacity channel that needs >50GHz spectrum
The CDC solution allows the operator to handle unpredictable A-Z services or temporary bandwidth demands over the full life of the network. Reconfigurations such as wavelength defragmentation and route optimization are also made possible to scale the network for support of more services. CDC also supports the transport of Super Channels when these become available.
CDC can operate with a photonic control plane for increased automation of operations as well as to support automated photonic restoration and other future capabilities.
Gridless networks are the evolution of photonic line systems to improve spectral efficiency and flexibilities
Channel grid is no longer required to be centered at ITU wavelengths/frequencies.
Question 67: Why Do We Need Gridless?
▪ To get improved spectral efficiency with existing 40G/100G technology.
▪ Define a super-channel that has multiple sub-channels within it, in order to fit the same channels in a smaller region of the spectrum.
▪ Support higher line-rate transponders.
▪ In order to get the same reach/performance from 400 Gb/s and 1 Tb/s transponders, we have no choice but to increase the spectral width of these signals well beyond 50GHz or even 100GHz spacing.
Question 68: What Is Coherent Communication?
Definition of coherent light
A coherent light consists of two light waves that:
1) Have the same oscillation direction.
2) Have the same oscillation frequency.
3) Have the same phase or maintain a constant phase relationship with each other. Two coherent light waves produce interference within the area where they meet.
Principles of Coherent Communication
Coherent communication technologies mainly include coherent modulation and coherent detection.
Coherent modulation uses the signals that are propagated to change the frequencies, phases, and amplitudes of optical carriers. (Intensity modulation only changes the strength of light.)
Modulation detection mixes the laser light generated by a local oscillator (LO) with the incoming signal light using an optical hybrid to produce an IF signal that maintains the constant frequency, phase, and amplitude relationships with the signal light.
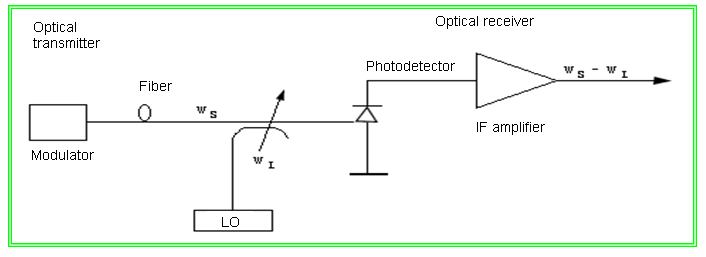
The two ideas behind using the coherent communication techniques are:-
First, the receiver sensitivity can be improved approximately by up to 20 dB compared with that of IM/DD systems.
Second, the use of coherent detection can allow a more efficient use of fiber bandwidth by increasing the spectral efficiency of WDM systems.
Question 69: Why heterodyne detection technique is used in Coherent technology receivers rather homodyne?
As we know that both homodyne or heterodyne detection can be used to convert the received optical signal into an electrical form. In case of homodyne detection, the optical signal is demodulated directly to the baseband. Although simple in concept, homodyne detection is difficult to implement in practice, as it requires a local oscillator whose frequency matches the carrier frequency exactly and whose phase is locked to the incoming signal. Such a demodulation scheme is called synchronous and is essential for homodyne detection. Although optical phase-locked loops have been developed for this purpose, their use is complicated in practice.
Heterodyne detection simplifies the receiver design, as neither optical phase locking nor frequency matching of the local oscillator is required. However, the electrical signal oscillates rapidly at microwave frequencies and must be demodulated from the IF band to the baseband using techniques similar to those developed for microwave communication systems. Demodulation can be carried out either synchronously or asynchronously. Asynchronous demodulation is also called incoherent in the radio communication literature. In the optical communication literature, the term coherent detection is used in a wider sense.
A lightwave system is called coherent as long as it uses a local oscillator irrespective of the demodulation technique used to convert the IF signal to baseband frequencies.
* In case of homodyne coherent-detection technique, the local-oscillator frequency is selected to coincide with the signal-carrier frequency.
* In the case of heterodyne detection, the local-oscillator frequency is chosen to differ from the signal-carrier frequency.
Question 70: What does Tap ration means for splitter and Coupler?
Fiber splitters/couplers divide optical power from one common port to two or more split ports and combine all-optical power from the split ports to one common port (1 × N coupler). They operate across the entire band or bands such as C, L, or O bands. The three-port 1 × 2 tap is a splitter commonly used to access a small amount of signal power in a live fiber span for measurement or OSA analysis. Splitters are referred to by their splitting ratio, which is the power output of an individual split port divided by the total power output of all split ports. Popular splitting ratios are shown in the Table below; however, others are available. The equation below can be used to estimate the splitter insertion loss for a typical split port. Excess splitter loss adds to the port’s power division loss and is lost signal power due to the splitter properties. It typically varies between 0.1 to 2 dB, refer to manufacturer’s specifications for accurate values.
It should be noted that the splitter function is symmetrical.
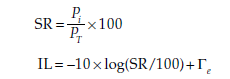
where IL = splitter insertion loss for the split port, dB
Pi = optical output power for single split port, mW
PT = total optical power output for all split ports, mW
SR = splitting ratio for the split port, %
Γe = splitter excess loss (typical range 0.1 to 2 dB), dB
Common splitter applications include
• Permanent installation in a fiber link as a tap with 2%|98% splitting ratio. This provides for access to live fiber signal power and OSA spectrum measurement without affecting fiber traffic. Commonly installed in DWDM amplifier systems.
• Video and CATV networks to distribute signals.
• Passive optical networks (PON).
• Fiber protection systems.
Example with calculation:
If a 0 dBm signal is launched into the common port of a 25% |75% splitter, then the two split ports, output power will be −6.2 and −1.5 dBm. However, if a 0 dBm signal is launched into the 25% split port, then the common port output power will be −6.2 dBm.
Calculation.

Launch power=0 dB=1mW
Tap is 25%|75%
so equivalent mW power which is linear will be
0.250mW|0.750mW
and after converting them ,dBm value will be
-6.02dBm| -1.24dBm
Some of the common split ratios and their equivalent Optical Power is available below for reference.
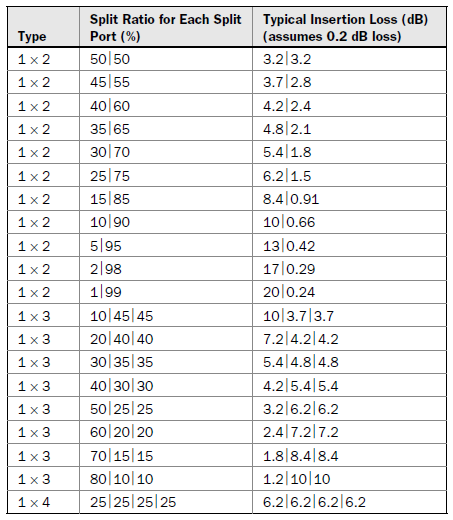
Question 71: What an OTDR can do for you?
The Optical Time Domain Reflectometer (OTDR) is useful for testing the integrity of fiber optic cables. An optical time-domain reflectometer (OTDR) is an opto-electronic instrument used to characterize an optical fiber. An OTDR is the optical equivalent of an electronic time -domain reflectometer. It injects a series of optical pulses into the fiber under test. It also extracts, from the same end of the fiber, light that is scattered (Rayleigh backscatter) or reflected back from points along the fiber. The strength of the return pulses is measured and integrated as a function of time, and plotted as a function of fiber length.
Using an OTDR, we can:
1. Measure the distance to a fusion splice, mechanical splice, connector, or significant bend in the fiber.
2. Measure the loss across a fusion splice, mechanical splice, connector, or significant bend in the fiber.
3. Measure the intrinsic loss due to mode-field diameter variations between two pieces of single-mode optical fiber connected by a splice or connector.
4. Determine the relative amount of offset and bending loss at a splice or connector joining two single-mode fibers.
5. Determine the physical offset at a splice or connector joining two pieces of single-mode fiber, when bending loss is insignificant.
6. Measure the optical return loss of discrete components, such as mechanical splices and connectors.
7. Measure the integrated return loss of a complete fiber-optic system.
8. Measure a fiber’s linearity, monitoring for such things as local mode-field pinch-off.
9. Measure the fiber slope, or fiber attenuation (typically expressed in dB/km).
10. Measure the link loss, or end-to-end loss of the fiber network.
11. Measure the relative numerical apertures of two fibers.
12. Make rudimentary measurements of a fiber’s chromatic dispersion.
13. Measure polarization mode dispersion.
14. Estimate the impact of reflections on transmitters and receivers in a fiber-optic system.
15. Provide active monitoring on live fiber-optic systems.
16. Compare previously installed waveforms to current traces.
Question 72: What is the difference between HD-FEC & SD-FEC in coherent transponders?
HD-FEC | SD-FEC | |
Definition | Decoding based on hard-bits(the output is quantized only to two levels) is called the “HD(hard-decision) decoding”, where each bit is considered definitely one or zero. | Decoding based on soft-bits(the output is quantized to more than two levels) is called the “SD(soft-decision) decoding”, where not only one or zero decision but also confidence information for the decision are provided. |
Application | Generally, for non-coherent detection optical systems, e.g., 10 Gbit/s, 40 Gbit/s, also for some coherent detection optical systems with higher OSNR | coherent detection optical systems, e.g., 100 Gbit/s,400 Gbit/s. |
Electronics Requirement | ADC(Analogue-to-Digital Converter) is not necessary in the receiver. | ADC is required in the receiver to provide soft information, e.g., coherent detection optical systems. |
specification | general FEC per [ITU-T G.975];super FEC per [ITU-T G.975.1]. | vendor specific |
typical scheme | Concatenated RS/BCH | LDPC(Low density parity check),TPC(Turbo product code) |
complexity | medium | high |
redundancy ratio | generally, 7% | around 20% |
NCG | about 5.6 dB for general FEC;>8.0 dB for super FEC. | >10.0 dB |
Example(If you asked your friend about traffic jam status on roads and he replies) | maybe fully jammed or free | 50-50 but I found other way free or less traffic |
Question 73: Why is it preferable to put attenuator/pad at the Receive end of Optical Module?
Few analogies proving the subject:-
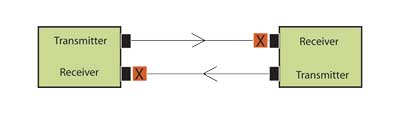
▪ If the distance is to short and the attenuator is too close to the transmitter, the reflected light off the attenuator will be directed back towards the Tx laser. Which will also blow your transmitter.so we place it at Rx.
▪ Also keeping attenuator at Rx will attenuate the noise along with the signal.
▪ The most important reason for putting them on the RX side is that you are protecting that which needs to be protected – the receiver in your optics. This way you know that you’re not going to potentially blow the receiver in your optics by plugging in too large a signal because you assumed there was an attenuator on the TX at the far end, and there wasn’t.
▪ It’s more convenient to test the receiver power before and after attenuation or while adjusting it with your power meter at the receiver, plus any reflectance will be attenuated on its path back to the source.
Keynote on Using Attenuators with Fiber Optic Data Links
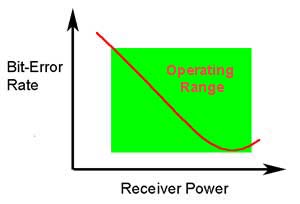
The ability of any fiber optic system to transmit data ultimately depends on the optical power at the receiver as shown above, which shows the data link bit error rate as a function of optical power at the receiver. (BER is the inverse of signal-to-noise ratio, e.g. high BER means poor signal to noise ratio.) Either too little or too much power will cause high bit error rates.
Too much power, and the receiver amplifier saturates, too little and noise becomes a problem as it interferes with the signal. This receiver power depends on two basic factors: how much power is launched into the fiber by the transmitter and how much is lost by attenuation in the optical fiber cable plant that connects the transmitter and receiver.
If the power is too high as it often is in short single mode systems with laser transmitters, you can reduce receiver power with an attenuator. Attenuators can be made by introducing an end gap between two fibers (gap loss), angular or lateral misalignment, poor fusion splicing (deliberately), inserting a neutral density filter or even stressing the fiber (usually by a serpentine holder or a mandrel wrap). Attenuators are available in models with variable attenuation or with fixed values from a few dB to 20 dB or more.
Gap-loss attenuators for multi mode fiber
Serpentine attenuators for single mode fiber
Generally, multimode systems do not need attenuators. Multimode sources, even VCSELs, rarely have enough power output to saturate receivers. Single mode systems, especially short links, often have too much power and need attenuators.
For a single mode application, especially analog CATV systems, the most important specification, after the correct loss value, is return loss or reflectance! Many types of attenuators (especially gap loss types) suffer from high reflectance, so they can adversely affect transmitters just like highly reflective connectors.
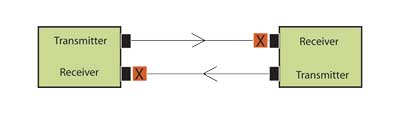
Choose a type of attenuator with good reflectance specifications and always install the attenuator ( X in the drawing) as shown at the receiver end of the link. This is because it’s more convenient to test the receiver power before and after attenuation or while adjusting it with your power meter at the receiver, plus any reflectance will be attenuated on its path back to the source.
Test the system power with the transmitter turned on and the attenuator installed at the receiver using a fiber optic power meter set to the system operating wavelength. Check to see the power is within the specified range for the receiver.
If the appropriate attenuator is not available, simply coil some patch cord around a pencil while measuring power with your fiber optic power meter, adding turns until the power is in the right range. Tape the coil and your system should work. This type of attenuator has no reflectance and is very low cost! The fiber/cable manufacturers may worry about the reliability of a cable subjected to such a small bend radius. You should probably replace it with another type of attenuator at some point, however.
Single mode attenuator made by wrapping fiber or simplex cable around a small mandrel. This will not work well with bend-insensitive fiber.
ref:http://www.thefoa.org/tech/ref/appln/attenuators.html
Question 74: How and where do we get pre and post FEC BER?
The first thing to note is that for each frame there are two sets of 20 parity bits. One set is associated with the end to end post FEC BER. The other is used to measure the span by span raw BER. The points at which these parity bits are terminated are illustrated below.
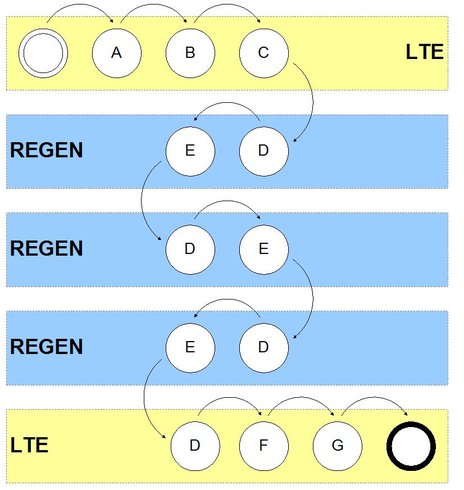
Processing point | Process description |
A | Calculate and insert the post FEC parity bits (those over which FEC is calculated) over the frame up to and including the MS OH. |
B | Encode FEC over the frame up to and including the MS OH. |
C | Calculate and insert the pre FEC parity bits (those over which FEC is not calculated) over the frame up to and including the RS OH. |
D | Terminate the raw BER based on the pre FEC parity bits. |
E | Re-calculate the pre FEC parity bits over the frame up to, and including, the RS OH. |
F | Decode FEC to produce the final data. |
G | Terminate the post FEC BER based on the post FEC parity bits. |
We can use the raw BER extracted at each RS terminating point (regens and LTEs) to estimate the post FEC BER. Note that this estimate is based on an assumption of a Poisson distribution of errors. In contrast the real post FEC BER can only be extracted at the MS terminating equipment (LTEs), and this is used to feed into the PM error counts.
Following are the terminologies you will come across when referring FEC Performance parameters:
PRE-FEC BER are the bit errors caused by attenuation, ageing, temperature changes of the optical fiber. PRE-FEC indicates that the signal on the optical fiber is FEC
encoded. The FEC decoder will recover the original signal, but depending on the PRE_FEC BER it will succeed to recover the original signal completely without errors.
Or, if the BER on the fiber is too high, the recovered signal will contain bit errors.
If the signal was FEC encoded the remaining bit errors after the decoder are called POST FEC BER.
The NO FEC BER are the bit errors detected when no FEC coding is used on the optical fiber.
Uncorrected words are the word that FEC is not able to corrects. It shows that the current FEC is not able to correct anymore and we need to look for more advance FEC.
Question 75: what are the Optical Fiber Link Design requirements?
he optical link design essentially is putting the various optical components, which we discussed earlier, so that information can be transmitted satisfactorily. The satisfactoriness of the transmission can be defined in terms of some characteristic parameters.
The user generally specifies the distance over which the information is to be sent and the data rate to be transmitted. The Designer then has to find the specification of the system components.
The designer generally has to define some additional criteria either as per the standards or as per the user specifications.
The Design criteria are given in the following.
Primary Design Criteria
◦ Data Rate
◦ Link length
Additional Design Parameters
▪ Modulation format e.g. Analog/digital
◦ Depends upon the type of signals user want to transmit. For example, if it is a TV signal, then may be analog transmission is more suited as it requires less bandwidth and better linearity. On the other hand, if data or sampled voice is to be transmitted, digital format may be more appropriate.
◦ The digital signals have to be further coded to suite the transmission medium and for error correction.
▪ System fidelity: BER, SNR
◦ The system fidelity defines the correctness of the data received at the receiver.
◦ For digital transmission it is measured by the Bit Error Ratio (BER) . The BER is defined as:-
◦
◦ In optical system, the BER has to be less than 10E-9
◦ For analog system, the quality parameter is the Signal-to-noise (SNR) ratio. In addition, there is a parameter called the inter-modulation distortion, which describes the linearity of the system.
▪ Cost : Components, installation, maintenance
◦ Cost is one of the important issues of the link design.
◦ The cost has three components, components, installation and maintenance.
◦ The component and the installations cost are the initial costs. Generally, the installation cost is much higher than the component cost for long links. This is especially true for laying the optical cable. It is therefore appropriate to lay the cables keeping in view the future needs.
◦ The optical link is supposed is supposed to work for at least 25years. The maintenance costs are as important as the initial cost. An initial cheaper system might end up into higher expenses in maintenance and therefore turn out to be more expensive as a whole.
▪ Upgradeability
◦ The optical fiber technology is changing very rapidly and the data rates are increasing steadily.
◦ The system should be able to adopt new technology, as well should be able to accommodate higher data rates with least possible changes.
▪ Commercial availability
◦ Depending upon which part of the world one is, the availability of the components and the systems may be an issue.
ref:http://nptel.ac.in/courses/117101054/16
Question 76: What are the major Difference between EDFAs requirements for Terrestrial (Land) Systems and Submarine Systems?
Compared with requirements for EDFAs for terrestrial applications and for Submarine applications, there are major important differences making the two types of amplifiers definitely two different components.
Terrestrial(Land) system | Submarine System |
•Reliability of land-based equipment is somewhat relaxed, corresponding to a 15-year required lifetime. | • Submarine systems are designed for a 25-year lifetime and a minimum of ship repair that imply reliability and redundancy of all the critical components. |
• Terrestrial equipment should enable operation over a wide temperature range of −5, +70°C (and −40, +85°C in storage conditions). This wide temperature range makes it necessary to implement cooling means for the highest temperatures and compensation means for temperature-sensitive devices. | • In submarine amplifiers, heat is dissipated from the outer side of the repeater container into the sea. Such a container is designed in order to make the heat go through the box from the pump device to the outer side, ensuring moderate temperature in all points. Temperature of the deep sea is indeed around +5°C. Specific care is taken for repeaters located at the coast or in shallow water, in order to guarantee no pump failure while avoiding Peltier cooling. For reliability reasons, no glue is used on the optical path. The constant temperature of the devices and the doped fiber incorporated in the amplifier makes it possible to perfectly tailor the gain spectrum of the submerged EDFAs, owing to very accurate equalizing filters and to concatenating hundreds of amplifiers. This would not be possible for land-based amplifiers whose gain cannot be guaranteed below 1 dB for a 30-nm bandwidth partly due to such temperature changes (while a few tenths of dB of gain excursion is reached for submarine amplifiers). |
• The infrastructure itself of terrestrial systems determines the actual characteristics of the amplifier that needs to cope with important variations of the span loss between two amplifier sites. In addition, for economic reasons, the amplifiers cannot be tailored to cope with this nonuniform link. | • In submarine systems, the link is manufactured at the same time as the amplifiers and much attention is paid to guarantee constant attenuation loss between amplifier values, while the amplifier has been designed to perfectly adapt to the link characteristics. |
• There are high gain range (20 to 35 dB) of the amplifiers incorporated in land-based systems and allowed by the margins given on the OSNR due to the reduced total link length. Gain equalizers therefore compensate for much larger gain excursion values than in submarine amplifiers and should therefore be located at amplifier midstage in order not to impact their equalizing loss on the amplifier output power. | • On the contrary, such filters can be placed after the single section of doped fiber that composes the amplifier in the case of submarine applications. |
Question 77: What is the relationship between BER and Q factor?
The Bit Error Rate (BER) of a digital optical receiver indicates the probability of an incorrect bit identification. In other words, the BER is the ratio of bits received in error to the total number of bits received. Below lists different values for BER and their corresponding errors per bits and over time.
As we know that, the photocurrent is converted to a voltage then measured. The measurement procedure involves a decision as to whether the bit received is a 1 or a 0. The BER is not only a function of the noise in the receiver and distortion in the system but also on the decision level voltage that is the threshold level above which the signal is classified as a 1 and below which the signal is classified as a 0. Even an ideal signal with no noise nor distortions has a non-zero BER if the decision level is set too high or too low. For example, if VD is set above the voltage of the 1 bit, the BER is 0.5, assuming equal probability of receiving a one and a zero.
BER | Error per 10E-15 bits | @ 10Gbps, One error in |
1×10-6 | 10,00,00,000 | 0.1 msec |
1×10-9 | 1,00,000 | 0.1 sec |
1×10-12 | 100 | 1.7 min |
1×10-15 | 1 | 1.2 days |
Mathematically, the Bit Error Rate is expressed as
BER = p(1)P(0 ⁄ 1) + p(0)P(1 ⁄ 0)
where p(1) and p(0) are the probabilities of receiving a 1 and a 0, respectively. P(0/1) is the probability of deciding a 0 when the bit is actually a 1, and P(1/0) is the probability of deciding a 1 when the bit is a 0.

Minimum BER as a function of Q
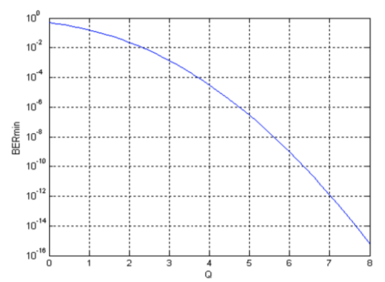
Question 78: What are the noise sources known in Optical fiber network?
Noise sources can be categorised as an active and passive.
Active sources such as optical plugs,lasers, receivers, and amplifiers generate noise in the fiber link.
Passive sources such as connectors, fiber, splices, and WDMs cause interference by distorting or reflecting the propagating signal power.
Below are ten major noise sources:
1. Signal-spontaneous noise (si-sp): This type of noise is generated by the signal mixing with amplified spontaneous emission noise generated in an optical amplifier. It is typically the dominant noise source in an amplified optical link. It is also known as amplified spontaneous emissions (ASE) noise.
2. Spontaneous-spontaneous noise (sp-sp): This type of noise is generated by ASE mixing with itself.
3. Shot noise (sh): This kind of electrical noise is generated by the receiver photodiode in both PIN and APD type receivers. APD type receivers have a better signal to noise ratio (SNR) due to their internal multiplication gain mechanism. Receiver noise level and minimum OSNR are established in transceiver design and cannot be controlled by system planning other than by selecting better transceivers.
4. Shot-spontaneous noise (sh-sp): This kind of noise is generated by shot noise added along-with ASE noise in the receiver. It is accounted for the transceiver’s OSNR specifications.
5. Thermal noise (th): This type of noise is generated by the front end of the receiver diode due to thermal activity and is accounted for in the transceiver’s OSNR specifications. The RMS photodiode
6. Multiple path interference noise (MPI): This noise is generated by the signal reflecting multiple times in the fiber and interfering with itself. Reflections are due to Rayleigh scattering and other reflective events such as connectors and splices. It is typically a concern for high signal powers and in distributed Raman amplifiers with high gains. This noise source is also known as double Rayleigh scattering (DRS). To keep MPI noise to a minimum, use good-quality, and clean ultra-polish (UPC) or angle polish (AP) type fiber connectors. Fiber splices that show OTDR reflections should be re-spliced.
7. Source spontaneous emissions (SSE): This noise is due to spontaneous photon emissions during the lasing process. These random photons add to the laser’s amplitude and phase, causing noise.
8. Mode partition noise (MPN): This noise occurs because of random variations of individual laser modes even though the total laser output power remains constant. The noise is generated in fibers where the signal dispersion wavelength is not zero. The fluctuating modes travel at different group velocities due to chromatic dispersion, which results in mode desynchronization and adds receiver noise. MPN occurs for MLM lasers but can also occur for SLM lasers that have significantly large side nodes and where the side mode suppression ratio (SMSR) is less than 20 dB.
9. Cross talk noise: DWDM (dense wavelength-division multiplexing) can cause interference noise as channel cross talk. The signal from one WDM channel appears on another channel resulting in interference. This is because a WDM cannot provide total 100% channel isolation. Typical adjacent channel isolation is approximately 30 dB down from adjacent channel signal power. At this level, the interference is not significant in most systems. However, it can be a concern for high channel launch powers.
10. Nonlinear distortions: Fiber can also cause noise interference when high power signals interact with the fiber, resulting in nonlinear distortions such as four-wave mixing. Maintaining low signal power below recommended limits can help keep this interference to a minimal level.
Question 79: What is resolution bandwidth?
The optical spectrum analyzer (OSA) is the device typically used to measure OSNR. Signal and noise measurements are made over a specific spectral bandwidth Br , which is referred to as the OSA’s resolution bandwidth (RBW). The RBW filter acts as a bandpass filter allowing only the set amount of light spectrum to strike the OSA’s photodetector. The photodetector measures the average optical power in the spectral width. It cannot discriminate between two separate signals in the RBW spectrum. If there is more than one signal in RBW, it will treat and display them as one. Therefore, the ability of an OSA to display two closely spaced signals as two distinct signals is determined by the RBW setting. Typically, an OSA’s RBW range is adjustable between 10 and 0.01 nm with common settings of 1.0, 0.5, 0.1, and 0.05 nm.
Question 80: What is noise equivalent bandwidth?
Noise power on signal cannot be measured directly because it is overpowered by the signal itself. Therefore, the noise measurement is performed at both sides of the signal, i.e outside of the signal boundary, using the OSA’s noise equivalent bandwidth (NEB) filter. The results are then interpolated to determine the noise power at the signal wavelength. The NEB filter has a rectangular passband and provides a more accurate noise measurement than the standard RBW filter.
Question 81: How long does it needs to continue with a BER test to make sure that the circuit meets BER specifications?
The BER test needs to be ran till enough bits gets transmitted and results are statistically usable. Actually, the test is run for an infinite amount of time, transmitting an infinite number of bits, that results in the channel’s true BER. However, this is not practical, and thus a statistical method is used to determine the channel’s BER to a certain confidence level of factor. This confidence level is a measure of percentage which represents the probability the true BER is equal to or better than the test BER. Typical confidence levels are 95% or 99%.
Question 82: Why is the BER not easy to simulate/calculate?
For a given design at a BER (such as 10-12 and a line rate of OC-3, or 155 Mbps), the network would have one error in approximately ten days. It would take thousand days to record a steady-state BER value. e.g. to provide a BER of 1e−12, there can be a one-bit error per 1e12 bits. At a 1.25 Gbps bit rate, it will take around 800 seconds (13.3 minutes) to transmit/receive 1e12 bits. Therefore, measurements sufficient to ascertain the BER down to 1e−12 or lower (at a sufficient confidence level) will generally require transmission a greater number of bits than 1e12 bits. Computer simulations on a system model can also be performed to determine BER. However, computer simulations presently can typically run about 1e6 bits in about an hour. Many existing applications require BER performance that is down to a level of 1e−12 or lower (e.g., 1e−17). Therefore, measurements or computer simulations might impose unacceptable time constraints.
This is why BER calculations are quite difficult. On the other hand, Q-factor analysis is comparatively easy. Q is often measured in dB. The next question is how to calculate Q dynamically. This is done from OSNR.
In other words, Q is somewhat proportional to the OSNR. Generally, noise calculations are performed by optical spectrum analyzers (OSAs) or sampling oscilloscopes, and these measurements are carried over a particular measuring range of Bm. Typically, Bm is approximately 0.1 nm or 12.5 GHz for a given OSA. From equation, showing Q in dB in
terms of OSNR, it can be understood that if B0 < Bc, then OSNR (dB )> Q (dB). For practical designs OSNR(dB) > Q(dB), by at least 1–2 dB. Typically, while designing a high bitrate system, the margin at the receiver is approximately 2 dB, such that Q is about 2 dB smaller than OSNR (dB).
Question 83: What is ROADM? What problems ROADM can solve?
Traditional DWDM network was static network. In earlier DWDM networks, it was point to point or rings (with point to point). Every channel route was pre-defined. To add and drop any new channel, a lot of manual intervention required.
ROADM is Reconfigurable (also some vendor refers remotely reconfigurable) OADM where, reconfigurability is a very desirable attribute in an OADM. Reconfigurability refers to the ability to select the desired wavelengths to be dropped and added on the fly, as opposed to having to plan ahead and deploy appropriate equipment. This allows carriers to be flexible when planning their network and allows lightpaths to be set up and taken down dynamically as needed in the network.
A reconfigurable optical add/drop multiplexer (ROADM) can provide flexibility and reconfigurability for an optical transport network (OTN). Such capabilities enable network operators to quickly and flexibly respond to network changes, such as establishing new light paths or releasing existing light paths.
ROADM application scenarios include colored & directioned, colored & directionless, and colorless & directionless.
Question 84: What is TVSP, and what is its effect?
The signal transmission quality is not stable over a long period of time because of the polarization effects occurring along the propagation path. The Time-varying system performance (TVSP) is deduced from testbed experiments where the fluctuations of the Q-factor are measured over a prolonged period of time. From this measurement, a Gaussian distribution is fitted to the measurements in order to deduce the standard deviation (s) and the average (mean Q) of the Q-factor distribution.
Question 85: What are the main reasons behind the fluctuation of the Q factor?
The following effects are the main sources of Q-factor fluctuations:
PDL(polarization-dependent loss):
This corresponds to the dependence of the insertion loss of passive components to the signal state of polarization (SOP).
PHB (polarization hole burning):
This corresponds to the dependence of the optical amplifier gain to the signal SOP. The PHB is an effect that is significant in single-wavelength transmission since the degree of polarization (DOP) of a laser source is close to 100% unless a polarization scrambler is used. In WDM transmission systems, including a large number of wavelengths, however, the DOP of the optical stream is close to 0% due to the random distribution of the different wavelengths SOP. This effect becomes, therefore, negligible in a WDM transmission system.
PDG (polarization-dependent gain):
This corresponds to the dependence of the EDFA gain to the pump SOP. The PDG can be considered for EDFA as equivalent to the PDL for passive components, and the impact on the transmission quality is the same as the PDL.
PMD(polarization mode dispersion):
This corresponds to the dependence of the fiber refractive index on the signal SOP.
Question 86: What is Spectral Efficiency, and what is its role in coherent technologies?
SE is defined as the information capacity of a single channel (in bit/s) divided by the frequency spacing Δf (in Hz) between the carriers of the WDM comb:
SE = Rs log2(M) /Δf (1+r)
where Rs is the symbol rate, M is the number of constellation points of the modulation format, and r is the redundancy of the forward error correction (FEC) code, for example, r = 0.07 for an FEC with overhead (OH) equal to 7%
More the SE, more data can be transmitted in a fiber. The total system capacity (defined as the maximum information in bit/s that can be transmitted by the WDM comb) is obtained as the product between the SE and the available bandwidth. The maximization of the SE thus plays an important role in the maximization of the overall system capacity.
The total system capacity (defined as the maximum information in bit/s that can be transmitted by the WDM comb) is obtained as the product between the SE and the available bandwidth. The maximization of the SE thus plays an important role in the maximization of the overall system capacity.
In the past years, the SE of optical systems has significantly increased, mainly due to the advent of coherent-detection technologies, which enabled the use of high-order modulation formats based on polarization-division multiplexing (PDM) [2], such as PDM-QPSK (quadrature phase-shift keying), with M = 4, PDM-16QAM (quadrature-amplitude modulation), with M = 8, and PDM-64QAM, with M = 12. However, the use of high-order modulation formats requires a higher optical signal-to-noise ratio (OSNR), which may result in a significantly reduced achievable transmission distance.
Question 87: What is the basic behind increasing SE?
To increase the SE, and consequently, the overall system capacity, involves reducing the frequency spacing Δf between the WDM sub-carriers. Here normalized frequency spacing 𝛿f and symbol Rate Rs defined as
𝛿f =Δf /Rs
For ultimate spectral efficiency, WDM channel spacings are reduced until the optical spectra of neighboring channels start to overlap noticeably. But this imposes linear crosstalk between adjacent WDM channels and becomes a main source of degradation. An efficient countermeasure to limit the crosstalk is based on an accurate spectral shaping of each sub-channel of the WDM comb is known as “Nyquist-WDM,”
Where the transmission of PDM-QPSK WDM signals with channel spacing equal to the symbol rate
The technique has also been successfully applied to the generation and transmission of higher-order modulation formats, such as PDM-8QAM, PDM-16QAM, PDM-32QAM, and PDM-64QAM with frequency spacing values equal or very close to the symbol rate.
Depending on the normalized frequency spacing 𝛿f among the WDM channels, three different categories of Nyquist-WDM signaling, which are
• 𝛿f = 1 (i.e., Δf = Rs): Ideal Nyquist-WDM
• 𝛿f > 1 (i.e., Δf > Rs): Quasi-Nyquist-WDM
• 𝛿f < 1 (i.e., Δf < Rs): Super-Nyquist-WDM
Question 88: What is the main purpose of using Coherent Detection in a system?
The main purposes of coherent detection are
(i) to linearly recover the In-Phase (I) and Quadrature (Q) components of the incoming signal, and
(ii) to suppress or cancel the common mode noise.
Question 89: How does changing modulation improves the reach of a system?
This is because of the reduction of the minimal distance between two points of the constellation, which reduces the resilience to channel impairments. For instance, going from a PDM-QPSK up to a PDM-16QAM transmission doubles the data rate at the cost of an optical reach divided by a factor of 5.
Question 90: What are the key characteristics of optical amplifiers?
Key characteristics of optical amplifiers are gain, gain bandwidth, gain efficiency, noise, gain saturation, and polarisation sensitivity:
• Gain is defined the ratio of output power to input power (measured in decibels).
• Gain efficiency is defined as the gain as a function of input power (decibels per milliwatt).
• Bandwidth is a function of frequency, and as such, gain bandwidth is the range of frequencies over which the amplifier is effective.
• Gain saturation is the maximum output power of the amplifier, beyond which it cannot increase despite the input power increase.
• Noise is an inherent characteristic of amplifiers. In optical amplifiers, noise is due to the spontaneous emission of excited ions.
• Polarization sensitivity is the gain dependence of optical amplifiers on the polarization of the signal.
Question 91: What are the main issues associated with EDFA in a DWDM link?
Main issues associated with EDFA designs are as follows:
1. The first issue is the flat gain. As EDFAs do not amplify all wavelengths through them the same; thus, the gain is not exactly flat.
2. The second issue is the pump power-sharing. The pump power is shared by all wavelengths in the link. Therefore the more the wavelengths, the lesser power per wavelength will be available. However, as wavelengths can get drop but not added, or some wavelengths get lost due to failures, EDFAs will amplify few wavelengths more.
These two issues can be mitigated by properly engineering the WDM system and by dynamic gain control.
3. The third issue is not as simple and is addressed differently. When engineering a fiber-optic path, it should be remembered that optical noise sources are cumulative and that the ASE of EDFAs introduces noise that degrades the signal to noise ratio (SIN). Although a strong optical signal launched into the fiber could overcome this, near the zero-dispersion wavelength region, four-wave mixing would become dominant, and it would degrade the SIN ratio.
Question 92: What are the parameters associated with fibers in a link?
Following are the major parameters associated with fibers:
• Forward attenuation/km
• Backward attenuation/km (if asymmetric)
• Polarization mode dispersion
• Polarization-dependent loss (PDL)
• Dispersion (chromatic and material)
• Zero-dispersion wavelength
• Dispersion flatness over spectrum range
• Birefringence
• Cutoff wavelength.
Question 93: What are the parameters associated with optical light sources in a link?
Following are the major parameters associated with optical light sources:
• OCh output power
• OCh wavelength AQ
• Line spacing
• Cutoff A (tunable sources)
• Tunability speed (tunable sources)
• Spectral width (tunable sources)
• Line width (light sources)
• Modulation depth (modulated sources) • Bit rate (max-min) (modulated sources) •
• Source noise
• Dependency on bias
Question 94: What are the parameters associated with optical light receivers in a link?
Following are the major parameters associated with optical light receivers:-
• Minimum threshold optical power, minimum sensitivity
• Responsiveness per wavelength, AQ
• Wavelength discrimination
• Receiver bit rate (max-min)
• Min-max threshold level (one-zero)
• Dependency on one’s density
• Dependence on polarization
• Demodulation
• Receiver noise
• Dependency on bias
• Dependency on temperature
Question 95: How does temperature affects EDFA performance?
The temperature sensitivity of an erbium-doped fiber amplifier (EDFA) is approximately –0.023 dB/°C in the temperature range of 25 to 95°C.
The gain of an EDFA often falls as the temperature increases. Temperature sensitivity is generally due to the misalignments of the fi- ber couplers that are inherent to the design, but slight effects from absorption and changes in the indices of refractions may also contribute.
Reference :
C. Liu et al. “Temperature and Electromagnetic Effects on Erbium-Doped Fiber Amplifier Systems,” Optical Engineering, 37(7), pp. 2095–2100, July 1998.
Question 96: What are some ways to increase the capacity of an optical system?
1. increasing the signal’s frequency;
2. increasing the number of fibers;
3. increasing the number of channels;
4. increasing the modulation complexity.
The first option would require a proportional increase in bandwidth, while the other options would require the inclusion or replacement of equipment, resulting in higher cost, complexity, and power consumption.
Question 97: What is the significance of the eye diagram?
The quality of the transmitted signal can be analyzed at a glance through an eye diagram, .which is generated by superimposing repetitive samples of the PAM2 signal. The vertical eye-opening is related to the signal amplitude and signal-to-noise ratio (SNR). Higher the opening, better the SNR.
Question 98: What is an OTDR?
An OTDR is a test instrument that sends short light pulses down an optical fiber to determine the fiber’s characteristics, attenuation, and length. Testing needs OTDR at one end of the fiber for the test. At the other end of the fiber a good reflective fiber end is required, such as an open connector, to produce strong reflections for good readings,
OTDR launches short pulses into the fiber over three or four wavelengths (typically 1310, 1410, 1550, and 1625 nm) and measures the elapsed time between the reflected pulses
The total elapsed time measured for the reflected pulses must be divided by two in order to account for the light pulse traveling twice the fiber length. The fiber length is determined by the OTDR from the pulse delay.
Power values w.r.t Intensity and Distance graph is plotted, which gives information about the losses, reflection, splice, break etc.OTDR traces can help locate high-reflective events for repair.ORL can be measured with an ORL test set or an OTDR
If the end of the fiber provides a poor reflection such as with an angle connector or broken fiber, the fiber length is too long, or the loss is too high, then measurements using this method may not be possible.
Question 99: Why does FEC introduce latency?
As we know that to improve correction capability, more powerful and complex FEC codes must be used. However, the more complex the FEC codes are, the more time FEC decoding will take. This term “baud” originates from the French engineer Emile Baudot, who was the inventor of 5-bit teletype code. The Baud rate actually refers to the number of signal or symbol changes that occurs per second. A symbol is one of the several voltage, frequency, or phase changes.
Baudrate = bitrate/number of bits per symbol ;
signal bandwidth = baud rate;
Baud rate:
It is the rate symbols which are generated at the source and, to a first approximation, equals to the electronic bandwidth of the transmission system. The baud rate is an important technology-dependent system performance parameter. This parameter defines the optical bandwidth of the transceiver, and it specifies the minimum slot width required for the corresponding flow(s).
Baud rate/symbol rate/transmission rate for a physical layer protocol is the maximum possible number of times a signal can change its state from a logical 1 to logical 0 or or vice-versa per second. These states are usually voltage, frequency, optical intensity or phase. This can also be described as the number of symbols that can be transmitted in 1 second. The relationship between baud rate and bitrate is given as.
Bit rate = baud rate * number of bits / baud
The number of bits per baud is deduced from the existing modulation scheme. Here, we are assuming that the number of bits per baud is one, so, the baud rate is the exactly same as the bit rate.
The spectral-width of the wavelength in GHz is equal to the symbol rate in Gbaud measured at the 3 dB point or the point where the power is half of the peak. As the baud rate increases, the spectral-width of the channels will increases proportionally. The higher baud rates, therefore, are unable to increase spectral efficiency, though there can be exceptions to this rule where a higher baud rate better aligns with the available spectrum. Increasing wavelength capacity with the baud rate, has far less impact on reach than increasing it with higher-order modulation.
Higher baud rates, offer the best potential for reducing the cost per bit in Flexi-grid DWDM networks and also in point-to-point fixed grid networks, even though higher baud rates are not significant in 50 GHz fixed grid ROADM networks. Higher baud rates also requires all the components of the optical interface, including the DSP, photodetector and A/D converters and modulators, to support the higher bandwidth. This places a limit on the maximum baud rate that is achievable with a given set of technology and may increase the cost of the interfaces if more expensive components are required.
Question 101: What is dBm and what are the major conversions used in Optical Network?
dBm or decibel-milliwatt is an electrical power unit in decibel (dB), referenced to one milliwatt (mW).
The power in decibel-milliwatts (P(dBm)) is equal to 10 times base 10 logarithm of the power in milliwatts (P(mW)):
P(dBm) = 10 · log10( P(mW) / 1mW )
The power in milliwatts (P(mW)) is equal to 1mW times 10 raised by the power in decibel-milliwatts (P(dBm)) divided by 10:
P(mW) = 1mW · 10(P(dBm) / 10)
1 milliwatt is equal to 0 dBm:
1mW = 0dBm
1 watt is equal to 30dBm:
1W = 1000mW = 30dBm
How to convert mW to dBm
How to convert optical power in milliwatts(mW) to dBm.
The power in dBm is equal to the base10 logarithm of the power in milliwatts(mW):
P(dBm) = 10 · log10( P(mW) / 1mW )
For example: what is the power in dBm for power consumption of 100mW?
Solution:
P(dBm) = 10 · log10( 100mW / 1mW ) = 20dBm
How to convert dBm to mW
How to convert power in dBm to milliwatts (mW).
The power in milliwatts (P(mW)) is equal to 10 raised by the power in dBm (P(dBm)) divided by 10?
P(mW) = 1mW · 10(P(dBm) / 10)
For example: what is the power in milliwatts for power consumption of 20dBm?
Solution:
P(mW) = 1mW · 10(20dBm / 10) = 100mW
How to Convert Watt to dBm
How to convert power in watts (W) to dBm.
The power in dBm is equal to the base 10 logarithm of the power in watts (W) plus 30dB:
P(dBm) = 10 · log10( P(W) / 1W ) + 30
For example: what is the power in dBm for power consumption of 100W?
Solution:
P(dBm) = 10 · log10( 100W / 1W ) + 30 = 50dBm
How to convert dBm to Watt
How to convert power in dBm to watts (W).
The power in watts (P(W)) is equal to 10 raised by the power in dBm (P(dBm)) minus 30dB divided by 10:
P(W) = 1W · 10( (P(dBm) – 30) / 10)
For example: what is the power in watts for power consumption of 40dBm?
Solution:
P(W) = 1W · 10( (40dBm – 30) / 10) = 10W
How to convert dBW to dBm
How to convert power in dBW to dBm.
The power in dBm is equal to the base 10 logarithm of the power in watts (W):
P(dBm) = P(dBW) + 30
For example: what is the power in dBm for power consumption of 20dBW?
Solution:
P(dBm) = 20dBW + 30 = 50dBm
How to convert dBm to dBW
How to convert power in dBm to dBW.
The power in dBW (P(dBW)) is equal to 10 raised by the power in dBm (P(dBm)) divided by 10:
P(dBW) = P(dBm) – 30
For example: what is the power in watts for power consumption of 40dBm?
Solution:
P(dBW) = 40dBm – 30 = 10dBW
dBm to Watt, mW, dBW conversion table
Power (dBm) | Power (dBW) | Power (watt) | Power (mW) |
-100 dBm | -130 dBW | 0.1 pW | 0.0000000001 mW |
-90 dBm | -120 dBW | 1 pW | 0.000000001 mW |
-80 dBm | -110 dBW | 10 pW | 0.00000001 mW |
-70 dBm | -100 dBW | 100 pW | 0.0000001 mW |
-60 dBm | -90 dBW | 1 nW | 0.000001 mW |
-50 dBm | -80 dBW | 10 nW | 0.00001 mW |
-40 dBm | -70 dBW | 100 nW | 0.0001 mW |
-30 dBm | -60 dBW | 1 μW | 0.001 mW |
-20 dBm | -50 dBW | 10 μW | 0.01 mW |
-10 dBm | -40 dBW | 100 μW | 0.1 mW |
-1 dBm | -31 dBW | 794 μW | 0.794 mW |
0 dBm | -30 dBW | 1.000 mW | 1.000 mW |
1 dBm | -29 dBW | 1.259 mW | 1.259 mW |
10 dBm | -20 dBW | 10 mW | 10 mW |
20 dBm | -10 dBW | 100 mW | 100 mW |
30 dBm | 0 dBW | 1 W | 1000 mW |
40 dBm | 10 dBW | 10 W | 10000 mW |
50 dBm | 20 dBW | 100 W | 100000 mW |
60 dBm | 30 dBW | 1 kW | 1000000 mW |
70 dBm | 40 dBW | 10 kW | 10000000 mW |
80 dBm | 50 dBW | 100 kW | 100000000 mW |
90 dBm | 60 dBW | 1 MW | 1000000000 mW |
100 dBm | 70 dBW | 10 MW | 10000000000 mW |
Question 102: Which components and technology is used in ROADM?
Wavelength Selective Switches or WSS form the heart of ROADM. A WSS route wavelength channels between input port (s) to the output port(s), whereby the state of connectivity of the switch can be set on a wavelength by wavelength basis. WSS Modules are available in switch sizes ranging from 1×2 to 1×9, with some recent product announcements extending this to 1×20 to 1×23.
WSS Technologies:
1. MEMS
2. DLP (Digital Light Processing)
3. Liquid Crystals (LC) Cells
4. Liquid Crystals on Silicon (LCoS)
5. Hybrid ( LC+MEMS)
REFERENCES
1. Lightwave Technology Telecommunication Systems by Govind P. Agrawal
2. Undersea Fiber Communication Systems by Jose ́ Chesnoy
3. Fault Detectability in Dwdm By Stamatios V. Kartalopoulos
4. Enabling Technologies for High Spectral-Efficiency Coherent Optical Communication Networks by Xiang Zhou Chongjin Xie
5. Multiple Internet Resources
6. www.mapyourtech.com (my website)
ABOUT THE AUTHOR
Sanjay Yadav, is an enthusiastic Optical Fiber Communication Professional having experience in various optical industries dealing with products, services ,design and operations. He believes in sharing knowledge which can help young enthusiasts to grow in their professional career.
You can visit his work on www.mapyourtech.com and reach on feedback@mapyourtech.com