Fiber optics has revolutionized the way we transmit data, offering faster speeds and higher capacity than ever before. However, as with any powerful technology, there are significant safety considerations that must be taken into account to protect both personnel and equipment. This comprehensive guide provides an in-depth look at best practices for optical power safety in fiber optic communications.
Viewing Safety Measures
Directly viewing fiber ends or connector faces can be hazardous. It’s crucial to use only approved filtered or attenuating viewing aids to inspect these components. This protects the eyes from potentially harmful laser emissions that can cause irreversible damage.
Fiber End Management
Unterminated fiber ends, if left uncovered, can emit laser light that is not only a safety hazard but can also compromise the integrity of the optical system. When fibers are not being actively used, they should be covered with material suitable for the specific wavelength and power, such as a splice protector or tape. This precaution ensures that sharp ends are not exposed, and the fiber ends are not readily visible, minimizing the risk of accidental exposure.
The Crucial Role of Cleaning
Optical connectors must be kept clean, especially in high-power systems. Contaminants can lead to the fiber-fuse phenomenon, where high temperatures and bright white light propagate down the fiber, creating a safety hazard. Before any power is applied, ensure that all fiber ends are free from contaminants.
Managing Connector and Splice Loss
Even a small amount of loss at connectors or splices can lead to a significant increase in temperature, particularly in high-power systems. Choosing the right connectors and managing splices carefully can prevent local heating that might otherwise escalate to system damage.
Ribbon Fibers and Test Cords
Ribbon fibers, when cleaved as a unit, can present a higher hazard level than single fibers. They should not be cleaved or spliced as an unseparated ribbon unless explicitly authorized. When using optical test cords, always connect the optical power source last and disconnect it first to avoid any inadvertent exposure to active laser light.
Avoiding Excessive Bending
Fiber optics are delicate and can be damaged by excessive bending, which not only risks mechanical failure but also creates potential hotspots in high-power transmission. Careful routing and handling of fibers to avoid low-radius bends are essential best practices.
Handling Board Extenders and Routine Maintenance
Board extenders should never be used with optical transmitter or amplifier cards. Only perform maintenance tasks in accordance with the procedures approved by the operating organization to avoid unintended system alterations that could lead to safety issues.
Test Equipment Usage
Employ test equipment that is appropriate for the task at hand. Using equipment with a power rating higher than necessary can introduce unnecessary risk. Ensure that the class of the test equipment matches the hazard level of the location where it’s being used.
System Modifications and Key Control
Unauthorized modifications to optical fiber communication systems or related equipment are strictly prohibited, as they can introduce unforeseen hazards. Additionally, key control for equipment should be managed by a responsible individual to ensure the safe and proper use of all devices.
Labeling and Signage
Optical safety labels are a critical aspect of safety. Any damaged or missing labels should be reported immediately. Warning signs should be posted in areas exceeding hazard level 1M, and even in lower classification locations, signs can provide an additional layer of safety.
Alarm Systems
Pay close attention to system alarms, particularly those indicating issues with automatic power reduction (APR) or other safety mechanisms. Prompt response to alarms can prevent minor issues from escalating into major safety concerns.
Raman Amplified Systems: A Special Note
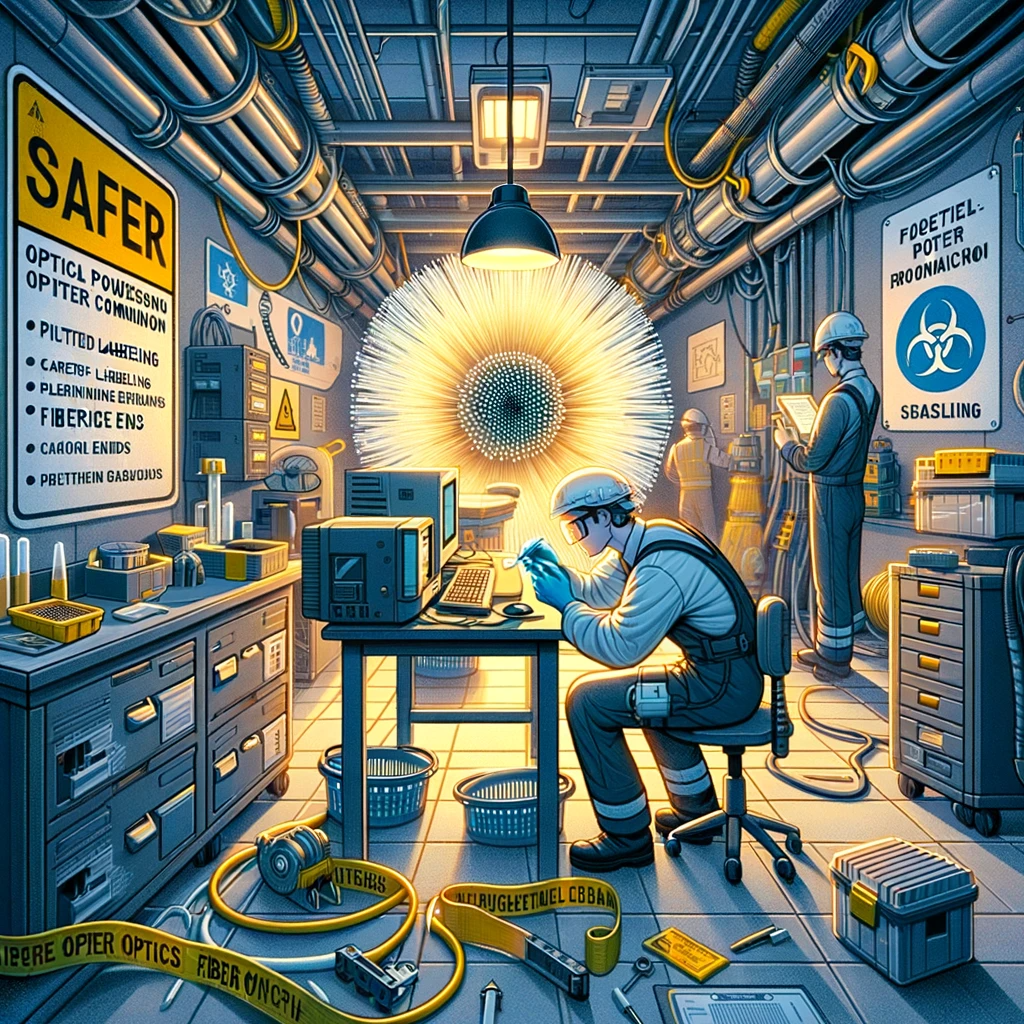
Raman amplified systems operate at sufficiently high powers that can cause damage to fibre or other components. This is somewhat described in clauses 14.2 and 14.5, but some additional guidance follows:
Before activating the Raman power
– Calculate the distance to where the power is reduced to less than 150 mW.
– If possible, inspect any splicing enclosures within that distance. If tight bends, e.g., less than 20mm diameter, are seen, try to remove or relieve the bend, or choose other fibres.
– If inspection is not possible, a high resolution OTDR might be used to identify sources of bend or connector loss that could lead to damage under high power.
– If connectors are used, it should be verified that the ends are very clean. Metallic contaminants are particularly prone to causing damage. Fusion splices are considered to be the least subject to damage.
While activating Raman power
In some cases, it may be possible to monitor the reflected light at the source as the Raman pump power is increased. If the plot of reflected power versus injected power shows a non‑linear characteristic, there could be a reflective site that is subject to damage. Other sites subject to damage, such as tight bends in which the coating absorbs the optical power, may be present without showing a clear signal in the reflected power versus injected power curve.
Operating considerations
If there is a reduction in the amplification level over time, it could be due to a reduced pump power or due to a loss increase induced by some slow damage mechanism such as at a connector interface. Simply increasing the pump power to restore the signal could lead to even more damage or catastrophic failure.
The mechanism for fibre failure in bending is that light escapes from the cladding and some is absorbed by the coating, which results in local heating and thermal reactions. These reactions tend to increase the absorption and thus increase the heating. When a carbon layer is formed, there is a runaway thermal reaction that produces enough heat to melt the fibre, which then goes into a kinked state that blocks all optical power. Thus, there will be very little change in the transmission characteristics induced by a damaging process until the actual failure occurs. If the fibre is unbuffered, there is a flash at the moment of failure which is self-extinguishing because the coating is gone very quickly. A buffered fibre could produce more flames, depending on the material. For unbuffered fibre, sub-critical damage is evidenced by a colouring of the coating at the apex of the bend.
Conclusion
By following these best practices for optical power safety, professionals working with fiber optic systems can ensure a safe working environment while maintaining the integrity and performance of the communication systems they manage.
For those tasked with the maintenance and operation of fiber optic systems, this guide serves as a critical resource, outlining the necessary precautions to ensure safety in the workplace. As the technology evolves, so too must our commitment to maintaining stringent safety standards in the dynamic field of fiber optic communications.